Diabetes is an increasing health problem that could increase the rate of congenital malformations. Therefore, the burden on health care providers and society is increasing, which could be mitigated by providing treatments that are based on natural, available, and cheap materials. One of the natural hypoglycemic treatments that had been used is Corn Silk aqueous extract (CSE). One of the targeted beneficiaries from these treatments are diabetic pregnant women. The aim is to study the teratogenicity of CSE, as well as whether it reduces the effect of hyperglycemia on the offspring. Female mice were divided into four groups: Control, Treated, Diabetic Control, and Diabetic Treated. Pregnant mice of treated groups were given a daily dose of 4 g/kg of CSE orally, while control mice were given equal amounts of distilled water. Samples were collected on day 16.5 of pregnancy, birth (neonates), and 3 weeks postnatal. Whole body weight, length, and morphological malformations were recorded. The most frequent malformation observed was Intrauterine Growth Retardation (IUGR) among the diabetic groups’ samples. The rate of malformations in fetuses of diabetic mothers was not decreased, but in 3-week-old mice of treated mothers we observed a significant increase in whole body weight and length. We concluded that CSE consumption during pregnancy might not be favorable in the dose used, while it might have some benefits if used while nursing. More studies with different doses and their frequencies are needed. Also, the effect of the extract on lactation and nursing milk composition should be investigated.
The effect of corn silk, as a hypoglycemic, on the congenital malformations caused by diabetes in mice: A morphological study
Dema Alsudairi, Fatma Al-Qudsi
Biological sciences department, science faculty, King Abdulaziz University
SUMMARY
Sign up or Login
INTRODUCTION
Diabetes is an increasingly spreading disease all over the world (El-Abhar and Schaalan, 2014; International Diabetes Federation, 2017). Diabetes mellitus is classified into three main types. Type 1 diabetes mellitus (T1DM), which is characterized by the absence of insulin secretion due to the destruction of β-cells by the immune system or idiopathic reasons. Type 2 diabetes mellitus (T2DM), which is caused by insulin resistance and/or insufficient insulin production. Gestational Diabetes mellitus (GDM) is diabetes that begins during pregnancy (Chen, 2005). Women with GDM may be undiagnosed type 2 diabetics (Correa et al., 2008). Diabetes affects 2-5% of pregnant women. In 2013, more than 21 million diabetic pregnancies were reported. Maternal diabetes during pregnancy is either Pre-gestational (PGDM), type 1 or 2 existing before pregnancy, or Gestational (GDM), that occurs in mid gestation. 87.5% of diabetic pregnancies are GDM, while the remaining 12.5% result from PGDM (Dowling et al., 2014; Wahabi et al., 2017).
Saudi Arabia is named by International Diabetes Federation among the highest 10 countries in diabetes prevalence. It is also the highest among the Middle East countries (Lasheen et al., 2014; Wahabi et al., 2014). The Saudi Ministry of Health reported 0.9 million diabetic patients in 1992, compared to 2.5 million in 2010. This rapid increase in around 2 decades raised concern (Alotaibi et al., 2017). In 2014, 13.9% of the health expenditure was spent on the diabetic population in Saudi Arabia (Alwin Robert et al., 2017). Consequently, the burden on health care providers and society is increasing, which could be mitigated by providing treatments that are based on natural, available, and cheap materials. One of the targeted beneficiaries from these treatments are diabetic pregnant women.
Maternal hyperglycemia is associated with risk outcomes for the mother, fetus, child, and adult offspring. For the mother, there is an increased risk for premature and C-section delivery due to large sized infant, and higher possibility of developing gestational diabetes mellitus (GDM) in later pregnancies, and type 2 diabetes mellitus (T2DM) later in life. The fetus could be large for gestational age, develop metabolic problems, higher long-term risk for diabetes and obesity, and female offspring susceptibility of GDM in the future due to fetal genome epigenetic modifications (Monteiro et al., 2016; Stewart and Murphy, 2015). The risks associated with DM includes: macrosomia, congenital malformations, stillbirth, preterm delivery and birth asphyxia (Wahabi et al., 2014). Diabetes has a teratogenic effect on multiple organ systems, most commonly: cardiovascular, musculoskeletal and central nervous system (Correa et al., 2008; Mills, 2010).
Corn Silk (CS) is one of the medicinal herbs traditionally used by the Chinese and the natives of the Americas, as well as in Serbia, Turkey, and France, for hyperglycemia and a variety of diseases. Stigma maydis or corn silk is the thread-like yellow-brown or light-green style/stigmas that make the Zea mays L. (corn) female flower. Corn silk (CS) is used to treat disorders associated with the urinary system including edema, kidney stones, cystitis, prostate disorders, bedwetting and urinary infections. It relaxes the bladder’s lining, increases the secretion of urine (due to its diuretic and kaliuretic properties (Velazquez et al., 2005), and reduces irritation. It is also used for gout, asthma, obesity and hypertension. CS possesses anti-depressant and anti-fatigue activity (Zhao et al., 2012), anti-fungal activity, and contains large amounts of antioxidants. The medicinal properties are attributed to its chemical compounds. It contains vitamins, proteins, carbohydrates, salts (Mg, K, Ca, and Na), volatile and fixed oils, alkaloids, steroids, tannins, saponins, and flavonoids (Guo et al., 2009; Hasanudin et al., 2012; Žilić et al., 2016). A toxicity study on mice done by Peng, Zhang, and Zhou, using three different doses of flavonoid-rich extract from CS (up to 10 g/Kg/day orally) for 28 days, showed that the extract has no toxicological effect or genotoxicity on either germ cells nor somatic cells. Therefore, it is safe to use it in the food industry and natural remedies (Peng et al., 2016).
In China, corn silk has been used as an antidiabetic treatment. Guo et al. (2009), studied the effect of CS extract on glycemic metabolism. They used 0.5, 1, 2, 4 g/kg body wt. doses of CS aqueous extract on alloxan-induced diabetic mice for 20 days. In mice groups which received the 2 and 4 g/kg doses, blood glucose levels decreased. They concluded that CS affected the glycemic metabolism via increasing insulin secretion and recovering damaged β-cells (Guo et al., 2009). In another study by Zhao et al. (2012), STZ-induced diabetic rats received 300, 400, 500 mg/kg body wt. of polysaccharides from CS extract (POCS) daily for 4 weeks. It had a hypoglycemic and hypolipidemic effect on diabetic rats, and no effect on normal ones (Zhao et al., 2012).
Maternal treatment with corn silk extract could reduce the pregestational diabetes teratogenicity. This study will be focusing on the effect of Corn Silk aqueous Extract (CSE) on the fetus and offspring of diabetic and non-diabetic pregnant mice.
MATERIALS AND METHODS
All procedures took place at King Fahad Medical Research Center (KFMRC) and were approved by the biomedical ethics research committee in King Abdulaziz University (KAU) (reference Number 345-19).
In this study, Swiss white rodless (SWR) adult male and female mice weighing 25-30 g, obtained from KFMRC, were used.
Female mice were distributed in cages, 5 in each cage, while each male was isolated in a cage. The animals were acclimated for a week in a room with 22±2oC, normal humidity and 12 hr. light/dark cycle, with free access to water and food (animal feed).
Corn silk (CS) was harvested from a local farm in Albudaiya (Duba, Tabuk Province, Saudi Arabia).
In this study, Streptozotocin was used to induce diabetes mellitus in experimental animals, as reviewed by Tripathi and Verma (2014). The used STZ (Sigma S0130-1G) was purchased form Bayouni Trading Co. LTD. Normal saline, formalin and diethyl ether were purchased from Al-Rowad Modern supply of medical equipment in Jeddah.
Preparation of corn silk extract
Corn silk (CS) was dried for a week in the farm, then transported to Jeddah. Corn silk was dried in a well-ventilated room for three weeks, then stored in a fridge (Fig. 1).
The method used to prepare the extract was based on previous studies (Velazquez et al., 2005; Guo et al., 2009) with slight modifications. First, the water extract was prepared by adding 100 ml of hot water to 10 g of dried CS and left for 20-30 min. Then the solution was filtered using filter paper (Whatman). The filtrate was frozen under -30oC in a deep freezer to prepare it for freeze-drying (lyophilization).
Lyophilization (Freeze-drying)
In order to measure exact amounts of the extract powder to prepare the doses, Freeze- Dryer (ilShinBioBase, Model FD8518) was used to dry (lyophilize) the extract. After drying, the samples were weighed and tightly sealed to be preserved in the freezer until needed. It was noticed that the dry extract absorbed water rapidly, so the water extract was placed in small plastic containers, which were placed in glass flasks.
Preparing the experimental solution
To prepare the dose freshly, we calculated the amount needed for each mouse (4 g/kg) and dissolved the extract powder in 0.5 ml of distilled water per mouse.
Working plan
The animals were divided into two main groups: non-diabetic and diabetic. Each group was divided into control and treated.
Group 1: Non-diabetic control (C), were given distilled water. Group 2: Non-diabetic treated (T), were given corn silk extract. Group 3: Diabetic control (DC), were given distilled water. Group 4: Diabetic treated (DT), were given corn silk extract.
Diabetes induction
Female mice were injected intraperitoneally with 75 mg/kg of STZ dissolved in cold normal saline, for three consecutive days (Martin et al., 2004; Deeds et al., 2011; Dowling et al., 2014). The dose was calculated and dissolved in 0.2 ml of normal saline for each mouse, making sure that the administration took place within 15 mins after preparation (STZ has a half-life of 15 min. and is cleared from the blood stream rapidly). After 10-14 days, blood glucose levels of the injected mice were checked using accu-check Performa (Roche Diabetes Care), if blood glucose levels were >200 mg/dL mice were considered diabetic (Loeken, 2005; Jawerbaum and White, 2010; Mostafa et al., 2014; Dong et al., 2015; Wang, Reece and Yang, 2015).
Mating
The estrus stages of diabetic and nondiabetic females were checked to determine readiness for mating (Caligioni, 2009; Byers et al., 2012). Females in the estrus and proestrus stages were gathered with males; 2 females with 1 male and left for 24 h., then checked in the next morning for vaginal plugs. Once the vaginal plug was seen, Embryonic day 0.5 of pregnancy (E0.5) would be established, so blood glucose and weight were recorded, and dose administration began.
Dose administration
Starting at E0.5, the mothers in the treated groups were given a dose of 4 g/kg CSE dissolved in 0.5 ml of distilled water, orally using a gavage (24G x 1” animal feeding needle, purchased from Pet Surgical), five days a week. Mothers in the control groups were given equal amounts of distilled water (0.5 ml each).
Sample collection
At the age, E16.5, two mothers of each group were dissected. The uterus was taken out to extract the fetuses. Each fetus was numbered, photographed and weighed, then preserved in 10% formalin.
The neonates and 3-week-old offspring were numbered, photographed and weighed, then euthanized (using diethyl ether) to be preserved in 10% formalin.
For 3-week samples, the numbers were reduced especially in DC and DT groups due to death during lactation period (from birth till three weeks).
Photography
During dissection and sample collection videos and still photos were taken using a mobile (iPhone 6S) supported by a tripod. The distance and zooming were unified for each age treatment.
The preserved samples (E16.5 and neonates) were photographed under a dissecting microscope (Olympus SZX10) using an iPhone 6S held using an iPhone adapter (iDu Optics LabCam Microscope adapter for iPhone 6/6S) purchased from Amazon.com.
Morphology
The morphology of the control fetuses, neonates and 3-week-old offspring was examined and compared to the controls in previous literature (Theiler, 2013). Morphological malformations were recorded in all groups, including IUGR comparing sample weights to the lowest weight of control fetuses.
Morphometry
The photos were used for morphometrics using the image tool program, accessed and downloaded in 2019 from (http://cme.msu.edu/cmeias/). The whole length, starting from the tip of the nose to the beginning of the tail, as well as tail length were measured, in addition to placental diameters in E16.5 fetuses. (Fig. 2).
Statistical analysis
The measurements and weights were statistically analyzed using SPSS program (IBM SPSS Statistics, v. 1.0.0.1275). Data were analyzed with One-way ANOVA, then Two-independent sample test (Mann-Whitney) was done to test the significance of differences between groups.
RESULTS
Morphology of E16.5 mouse fetus
Normal 16.5-day mouse fetuses were covered by wrinkled, transparent skin which subcutaneous veins could be seen through. The four limbs and tail were clearly seen. The eyes were covered with fused eyelids. The auricle of the ear was well-defined. The upper section of the back was straight. The snout had clear mouth and nose. The neck was too short, almost absent. Hair follicles could be seen all over the body and in an area in the snout where whiskers will grow (Fig. 3A, B). One control case was seen where the placentas of two adjacent fetuses were attached, although each had its own amnion sac (Fig. 3C, D).
Fetuses in all groups seemed similar to the control group. In the T group, fetuses were similar to the ones in the control groups, and no obvious malformations were observed.
However, some malformations were noticed in some groups. In the diabetic control group, a fetus had an exposed brain (Exencephaly) where the cerebral cortex and the cerebellum could be seen (Fig. 4G, H). Another fetus from the same group had underdeveloped auricle. In the diabetic treated group, a fetus had an exposed brain (exencephaly) with bleeding parts (Fig. 3I, J). Another fetus from the same group had an extreme case of IUGR, in which it seemed small, with short limbs, and had thin and clear skin (Fig. 3K, L).
Morphology of neonate mice
Normal newborn mice in this study had a well-defined body, a tail, and four limbs with clear digits. The skin, with visible hair follicles, covered the body. Stomach filled with milk could be seen through the skin from the left side, and the dark color of the liver could be seen from the right side. In the head, eyes could be seen through the closed eyelids, ears were closed, and a snout with clear nasal openings and whiskers was visible. The neck was thick and short (Fig. 4A).
Neonates in all groups seemed similar to the control group. However, some malformations were noticed in different groups. In the treated group, one had an open left eyelid. Among the diabetic control group, the snout of one fetus seemed long and slightly narrow. One from the diabetic treated group was small and had a malformation in the posterior end of the body, where the tail was very short, thin, and almost transparent (caudal growth defect) (figure 4D).
Morphology of 3-week-postnatal mice
The 3-week old mice were similar to adult mice, with four limbs, a tail, and skin with white hair. Fully developed eyes with open eyelids and open auricle were visible. Mice in all groups were similar to the control with variations in body size. Mice in the treated group seemed larger than the controls, while mice in the diabetic control group were smaller compared to the control group.
Morphometry results
The effect of CSE on the Whole-Body Weight of fetuses and offspring
In this study, the normal whole-body weight mean of mouse fetus was 0.89g at E16.5. Corn silk aqueous extract caused a very slight non-significant decrease in whole body weight (WBW) in the T group compared to the C group. Diabetes caused a significant decrease in WBW in DC group compared to C group (p=0.000). It also caused a significant WBW decrease (p=0.045) in DT group compared to T group. CSE caused a significant decrease in DT (p=0.034) compared to C group while it caused a slight non-significant increase in WBW compared to DC group (figure 6). All fetuses in the T group weighed more than 0.77 g (the lowest control weight). While the percentage of fetuses in the DC group weighing less than 0.77 g was 68.2%, and 52.4% in the DT group (Table 1).
The normal body weight mean of neonate mice in this study was 1.6035 g. Both CSE and diabetes caused significant decrease in neonates’ WBW in T and DC (p=0.002), and DT (p=0.003) groups compared to C group. Diabetes and CSE also caused slight non-significant decreases in WBW of DT group compared to T group, and DT compared to DC (Fig. 5). The lowest weight of control fetuses was (1.43 g). In the T group, 68.8% had IUGR, while it occurred in 53.8% and 66.7% of DC and DT fetuses respectively.
At 3 weeks of age, the normal WBW mean of mice in this study was 7.0733 g. There was a significant increase in WBW caused by CSE in T group compared to the C (p=0.000), DT group compared to C (p=0.001) and in DT compared to DC group (p=0.002). On the other hand, diabetes caused a significant decrease in WBW comparing DC with C group (p=0.000), and DT with T group (p=0.046) (Fig. 6).
The effect of CSE on the Whole-Body Length of fetuses and offspring
In this study, 16.5-day-old embryos’ normal body length was 3.4887 cm. Comparing the T with C group, there was a non-significant decrease in whole body length caused by CSE. In addition, there was a non-significant decrease comparing DT to C. Body length significantly decreased due to diabetes in the DC group compared to C group (p=0.000). Diabetes also caused a non-significant decrease in DT compared to T. In the diabetic groups, CSE caused a non-significant increase comparing DT with DC (Fig. 5).
Between neonates, the normal mean body length was 4.0977 cm in this study. There was CSE-related significant decrease in body length when T was compared with C group (p=0.021) also comparing DT and DC group (p=0.014). Comparing DT to C group there was a significant decrease (p=0.004) also. On the other hand, the comparison between DC with C, and DT with T group showed non-significant decreases related to diabetes (Fig. 5).
In this study, 8.108 cm was the normal mean body length for 3-week-old mice offspring. Comparing between T and C groups a significant increase in body length was associated with CSE (p=0.001), also in DT with DC (p=0.001) however DT group was slightly non significantly higher compared to C, while diabetes caused significant decreases in DC compared to C (p=0.001), and DT compared to T (p=0.007) (Fig. 6).
The effect of CSE on the Tail Length of fetuses and offspring
In this study in 16.5-day-old fetuses, the mean of normal tail lengths was 0.9643 cm. Among the groups of this age, CSE caused a significant decrease in tail length comparing T and C groups (p=0.001), but caused a non-significant increase comparing the diabetic groups DT with DC. Comparing DT to C (p=0.000), there was a significant decrease in the length of tails. The mean tail length in the DC was significantly decreased by diabetes compared to C group (p=0.000). Diabetes also caused a significant decrease in DT group tail length compared in T group (p=0.009) (Fig. 5).
The normal mean tail length of neonates in this study was 1.2175 cm. CSE caused a slight non-significant decrease in tail length in the T and DT groups compared to C. It also caused a significant decrease in DT compared to DC (p=0.027). Comparing DC with C, diabetes caused a significant increase in tail length (p=0.032). Diabetes caused a non- significant decrease among the diabetic groups DT and T (Fig. 5).
In 3-week-old mice offspring of this study, the normal mean tail length was 4.6227 cm. The treated group had significantly longer tails than the control group caused by CSE (p=0.000). However, DT group mice had longer tails, which were not significantly different compared to the controls. Also, CSE caused a significant increase in tail length in DT compared to DC (p=0.011). Comparing DC with C, diabetes caused a significant decrease in tail length (p=0.003). Diabetes also caused a significant decrease in tail length in DT compared to T (p=0.001) (Fig. 6).
The proportion of tail length to whole body length was reduced in all experimental groups in 16.5 fetuses compared to C. This reduction was significant in DC (p=0.003) and DT (p=0.001) groups compared to the controls. In neonates this proportion had a very slight non-significant increase in T and DC groups, while it stayed non significantly reduced in DT group compared to the controls. In 3-week-old offspring, CSE caused a significant increase in the proportion of tail length to whole body length in T group (p=0.00), while there was a non-significant increase in DT compared to C. Diabetes clearly caused a significant decrease in DC (p=0.00) compared to C group.
The effect of CSE on placental diameter of fetuses
In this study, the mean of normal placenta diameter of E16.5 embryos was 0.695 cm. Compared to the control group of this age, CSE caused a significant increase in the T group placental diameter (p=0.008) and DT group (p=0.001). Diabetes caused a significant increase in the diameter of the placenta of DC compared to C group (p=0.004). It also non- significantly increased DT placental diameter compared to T group. CSE had non- significantly increased the placenta diameter in DT compared to DC (Fig. 5).
It was also noticed that the number of neonates and 3-week-old mice was reduced in the diabetic groups. The rate of major malformations observed on our study are listed in Table 1.
DISCUSSION
Diabetes mellitus during pregnancy is considered a teratogen that increases the rate of birth defects in humans and animal models (Savion et al., 2004). In this study, female mice were used as a model for diabetes mellitus during pregnancy to study the effect of corn silk extract, as a hypoglycemic agent, on fetal development with and without diabetes. It was noticed that diabetes in general seemed to decrease growth in postnatal, natal, and prenatal mice. On the other hand, the effect of CSE on growth varied between age groups, as growth was reduced during in 16.5 fetuses and neonates, while it was significantly increased in 3 weeks offspring. It seems that diabetes and CSE increased the diameters of the placenta of E16.5 fetuses. Few morphological anomalies were observed in different groups.
In this study, the main malformation seen in the DC group was Intrauterine Growth Retardation (IUGR). The CSE seemed to decrease the amount of IUGR as seen from the morphometric results. However, CSE was not able to get rid of IUGR completely, or reduce the congenital malformations seen as exencephaly. On the other hand, CSE seemed to significantly boost the growth of T and DT 3-week-old progeny compared to C and DC groups.
Many factors could affect the weight of the fetus, including: hormones, genetic, nutrition, placental, and growth factors (Li et al., 2015) and could play a role in IUGR. As Padmanabhan and Shafiullah (2001) reviewed in their literature, earlier studies showed an association between maternal diabetes and IUGR, a number of malformations, and increased placental weight. Anomalous changes in metabolism, fuel, and fuel-related compounds in diabetic mothers’ pregnancies (such as elevated glucose levels, ketones, and free radicals among others) may affect fetal growth and could cause congenital defects. The rate of Macrosomia incidents is higher in GDM, since hyperglycemia affects the fetus in the mid-gestation period, leading to fetal hyperglycemia and consequent abnormal fetal weight gain. IUGR, on the other hand, is more frequent in the case of PGDM, where hyperglycemia affects the embryo in the preimplantation stages. In that case, glucose receptors formation might be reduced by DM in the blastula, causing insufficient energy supply. It also increases apoptosis, leading to a reduction in cell number, which contribute in manifestation of birth defects including IUGR or embryonic death (Padmanabhan and Shafiullah, 2001).
From the results of this study, CSE caused a slight decrease in WBW and WBL in the treated group compared to controls in E16.5 fetuses and a significant decrease in neonates.
Although studies showed that CSE did not cause hypoglycemia to normal mice (Zhao et al., 2012), it might be that giving the normal pregnant females daily doses of CSE might have reduced their blood sugar temporarily, thus exposing embryos to low glucose supply for a short period environment for a short period, might have caused the reduced growth seen in the T group compared to the controls. On the other hand, CSE caused an increase in growth parameters in the diabetic treated group compared to the diabetic controls in E16.5 fetuses but a slight insignificant decrease in neonates. The proportion of tail length to body length followed the WBW and WBL pattern. This shows that CSE could have a slight role in reducing the effect of diabetes on fetuses and neonate growth. DM caused a decrease in growth in all ages: this decrease was significant except WBL in neonates. This result is contestant with previous studies (Kappen et al., 2011).
The placenta plays a vital role in providing the fetus in subsequent and stable oxygen and nutrient supply. Changes in placental dimensions might lead to instability in blood and nutrient supply to fetuses (Moore et al., 2018). Many factors might lead to an increase in placental diameter, causing instability in blood and nutrient supply.
In a study on the effect of diabetes on placental cellular composition, the weight of placentas from uncontrolled diabetic pregnancy were elevated compared to controlled diabetic and normal pregnancies. This elevation seemed to be due to more glycogen storage rather than increased cellularity, since the amounts of DNA and proteins were similar in all three groups (Husain et al., 2001). This is supported by Padmanabhan and Shafiullah’s (2001) study, in which they noticed that these changes are correlated with fetal growth retardation and other developmental delays.
In this study, both corn silk extract and diabetes caused an increase in placental diameters for E16.5 fetuses, even though growth parameters decreased. It seems that some factors present in CSE increased placental diameters, which might be caused by glycogen accumulation.
As for the 3-week-old progeny in this study, CSE significantly increased growth in both diabetic and nondiabetic treated groups, which enhanced the decrease that diabetes caused in the DC group. This could be due to increased mothers’ lactation or changing milk components. These results could lead to explore the benefits of using CSE extract in early life after diabetic pregnancy. More research is needed to explore these probabilities.
As mentioned in the non-diabetic groups results of E16.5 fetuses (C and T), CSE did not seem to affect the morphology of fetuses. On the other hand, among the DC and DT groups, each had one incident of a major malformation exencephaly, which is a neural tube defect that diabetes could induce (Loeken, 2005; Wilde et al., 2014). The fact that this defect was manifested in the diabetic groups only is consistent with earlier studies, which concluded that diabetes increased the rate of NTDs (Sugimura et al., 2009; Kappen et al., 2011), especially exencephaly (Bohuslavova et al., 2013). Loeken (2005) reviewed that hyperglycemia could induce embryonic oxidative stress, which inhibits the expression of Pax3 gene, which is important for neural tube closure. The exencephaly seen in DT group seemed different than the one seen in the DC group, as the head opening that the brain was protruding from seemed smaller.
In the neonates’ results of this study, an incident of caudal growth defect was manifested in the DT group, where the posterior end of the fetus was malformed (Fig. 5). This case could be named caudal regression, a NTD. It is a defect affecting the lower spine vertebrae ranging from agenesis to hypogenesis of the caudal vertebrae and associated parts. This defect is rare in normal pregnancies, but its rate increases 250 times in pregnancies affected by diabetes (Chan et al., 2002; Sugimura et al., 2009; Mills, 2010; Kappen, 2013). One cause could be oxidative stress, since maternal diabetes increases it. Oxidative stress induces DNA damage that activate DNA damage response (DDR) pathway during neurulation stage. Excess DNA damage leads to cell apoptosis (Dong et al., 2015). Another possible mechanism could be due to downregulation of a gene in the tail bud in response to retinoic acid, causing the malformation as reported by Chan et al. (2002). In their study, they injected diabetic and non-diabetic pregnant mice with two doses of retinoic acid (Vit. A), a known teratogen. They found that vitamin A significantly increased the vulnerability of fetuses of diabetic mothers to caudal regression compared to non-diabetic ones. They noticed that the amount of downregulation in low-dose diabetic mice is comparable to high-dose non-diabetics. This suggested that diabetes increases the amount of vitamin A transferred to the fetus (Chan et al., 2002). In this study, it seems that other factors might have affected the expression of the gene responsible for the tail formation.
To our knowledge, this is the first study on the effect of CSE on the fetuses and offspring. More studies should be done to detect the mechanisms of the CSE effect on Neural Tube (NT) closure. It seems that the CSE dose given to pregnant females in this study was not enough to antagonize the effect of DM as it was 4 g/kg a day for 5 days per week only.
Related articles


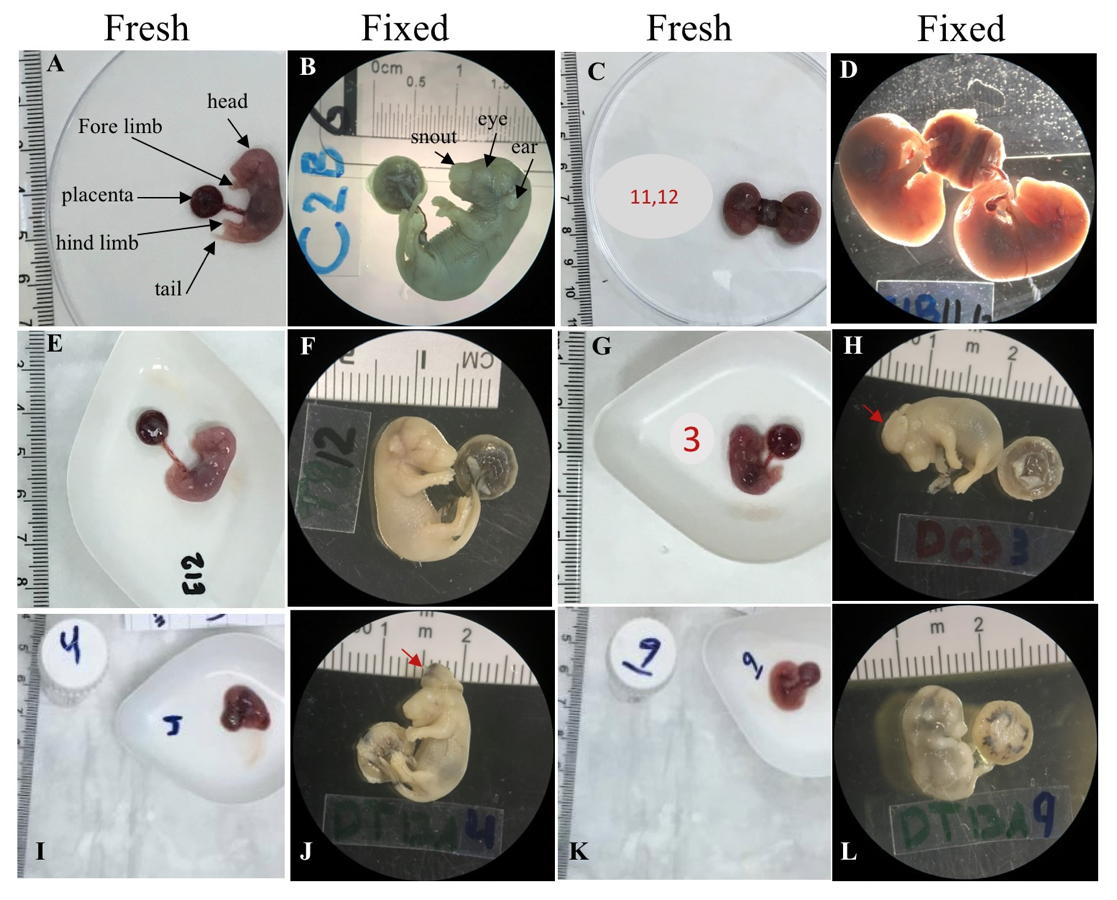

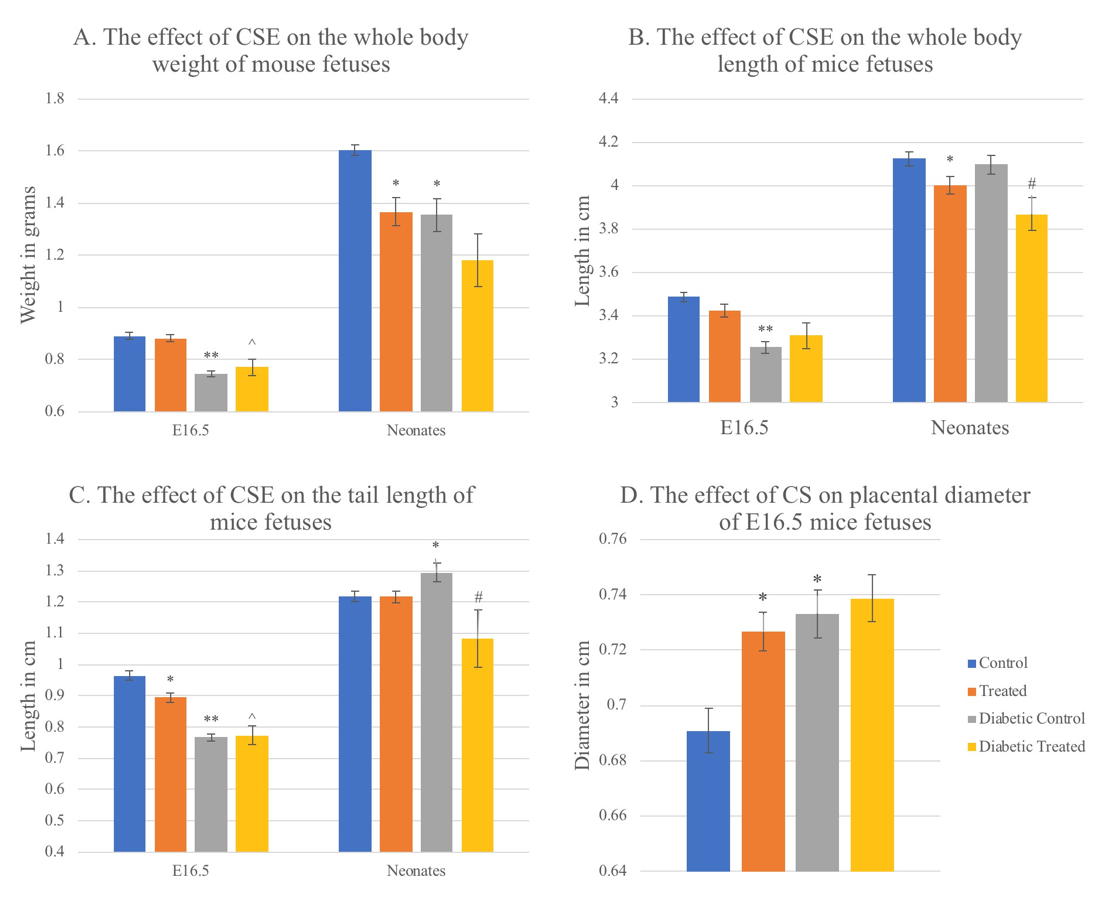
ALOTAIBI A, PERRY L, GHOLIZADEH L, AL-GANMI A (2017) Incidence and prevalence rates of diabetes mellitus in Saudi Arabia : An overview. J Epidemiol Glob Health, 7(4): 211-218.
ALWIN ROBERT A, AL DAWISH MA, BRAHAM R, MUSALLAM MA, AL HAYEK AA, AL KAHTANY NH (2017) Type 2 diabetes mellitus in Saudi Arabia: major challenges and possible solutions. Curr Diabetes Rev, 13(1): 59-64.
BOHUSLAVOVA R, SKVOROVA L, SEDMERA D, SEMENZA GL, PAVLINKOVA G (2013) Increased susceptibility of HIF-1α heterozygous-null mice to cardiovascular malformations associated with maternal diabetes. J Mol Cell Cardiol, 60: 129-141.
BYERS SL, WILES MV, DUNN SL, TAFT RA (2012) Mouse estrous cycle identification tool and images. PloS One, 7(4): e35538.
CALIGIONI CS (2009) Assessing reproductive status/stages in mice. Curr Protoc Neurosci, Appendix 4:Appendix 4I.
CHAN BWH, CHAN K-S, KOIDE T, YEUNG S-M, LEUNG MBW, COPP AJ, LOEKEN MR, SHIROISHI T, SHUM ASW (2002) Maternal diabetes increases the risk of caudal regression caused by retinoic acid. Diabetes, 51(9): 2811-2816.
CHEN C-P (2005) Congenital malformations associated with maternal diabetes. Taiwan J Obstet Gynecol, 44(1): 1-7.
CORREA A, GILBOA SM, BESSER LM, BOTTO LD, MOORE CA, HOBBS CA, CLEVES MA, RIEHLE-COLARUSSO TJ, WALLER DK, REECE EA (2008) Diabetes mellitus and birth defects. Am J Obstet Gynecol, 199(3): 237.e1-237.e9.
DEEDS MC, ANDERSON JM, ARMSTRONG AS, GASTINEAU DA, HIDDINGA HJ, JAHANGIR A, EBERHARDT NL, KUDVA YC (2011) Single dose streptozotocin-induced diabetes: considerations for study design in islet transplantation models. Lab Anim, 45(3): 131-140.
DONG D, YU J, WU Y, FU N, ARIAS VILLELA N, YANG P (2015) Maternal diabetes triggers DNA damage and DNA damage response in neurulation stage embryos through oxidative stress. Biochem Biophys Res Commun, 467(2): 407-412.
DOWLING D, CORRIGAN N, HORGAN S, WATSON CJ, BAUGH J, DOWNEY P, MCAULIFFE FM (2014) Cardiomyopathy in offspring of pregestational diabetic mouse pregnancy. J Diabetes Res, 2014: 624939.
EL-ABHAR HS, SCHAALAN MF (2014) Phytotherapy in diabetes: review on potential mechanistic perspectives. World J Diabetes, 5(2): 176-197.
GUO J, LIU T, HAN L, LIU Y (2009) The effects of corn silk on glycaemic metabolism. Nutr Metab, 6(1): 47.
HASANUDIN K, HASHIM P, MUSTAFA S (2012) Corn silk (Stigma maydis) in healthcare: a phytochemical and pharmacological review. Molecules, 17(8): 9697-9715.
HUSAIN SM, FROST R, MUGHAL ZM (2001) Effect of diabetes mellitus on rat placenta cellularity. Early Hum Dev, 60(3): 207-214.
INTERNATIONAL DIABETES FEDERATION (2017) IDF Diabetes Atlas. 8th edn. Brussels, International Diabetes Federation (IDF).
JAWERBAUM A, WHITE V (2010) Animal models in diabetes and pregnancy. Endocr Rev, 31(5): 680-701.
KAPPEN C, KRUGER C, MACGOWAN J, SALBAUM JM (2011) Maternal diet modulates the risk for neural tube defects in a mouse model of diabetic pregnancy. Reprod Toxicol, 31(1): 41-49.
KAPPEN C (2013) Modeling anterior development in mice: diet as modulator of risk for neural tube defects. Am J Med Genet C Sem Med Genet, 163C(4): 333-356.
LASHEEN AE, ABDELBASIT OB, SEIDAHMED MZ, HUSSEIN KA, MIQDAD AM, AL ZAHRANI MH, FARID GH, BADR HA (2014) Infants of diabetic mothers. A cohort study. Saudi Med J, 35(6): 572-577.
LI J, CHEN L, TANG Q, WU W, GU H, LIU L, WU J, JIANG H, DINGH, XIA Y, CHEN D, HUY, WANG X (2015) The role, mechanism and potentially novel biomarker of microRNA-17-92 cluster in macrosomia. Sci Rep, 5: 17212.
LOEKEN MR (2005) Current perspectives on the causes of neural tube defects resulting from diabetic pregnancy. Am J Med Genet C Sem Med Genet, 135C(1): 77-87.
MARTIN PM, ROON P, VAN ELLS TK, GANAPATHY V, SMITH SB (2004) Death of retinal neurons in streptozotocin-induced diabetic mice. Invest Ophthalmol Vis Sci, 45(9): 3330-3336.
MILLS JL (2010) Malformations in infants of diabetic mothers. Teratology 25:385-94. 1982. Birth Defects Res A Clin Mol Teratol, 88(10): 769-778.
MONTEIRO LJ, NORMAN JE, RICE GE, ILLANES SE (2016) Fetal programming and gestational diabetes mellitus. Placenta, 48 Suppl 1: S54-S60.
MOORE KL, PERSAUD TVN, TORCHIA MG (2018) The Developing Human-E-Book: Clinically Oriented Embryology. Elsevier Health Sciences.
MOSTAFA AM, MOHAMED WS, SERWAH AHA, SERWAH MA. (2014) Effect of diclofenac on plasma glucose level, insulin resistance, inflammatory markers and hepatocytes in diabetic albino rats. Egyp J Hosp Med, 15(54): 117-128.
PADMANABHAN R, SHAFIULLAH M (2001) Intrauterine growth retardation in experimental diabetes: possible role of the placenta. Arch Physiol Biochem, 109(3): 260-271.
PENG K-Z, ZHANG S-Y, ZHOU H-L (2016) Toxicological evaluation of the flavonoid-rich extract from Maydis stigma: Subchronic toxicity and genotoxicity studies in mice. J Ethnopharmacol, 192: 161-169.
SAVION S, GIDON-DABUSH S, FEIN A, TORCHINSKY A, TODER V (2004) Diabetes teratogenicity is accompanied by alterations in macrophages and T cell subpopulations in the uterus and lymphoid organs. Int Immunopharmacol, 4(10): 1319-1327.
STEWART ZA, MURPHY HR (2015) Gestational diabetes. Medicine, 43(1): 44-47.
SUGIMURA Y, MURASE T, OYAMA K, UCHIDA A, SATO N, HAYASAKA S, KANO Y, TAKAGISHI Y, HAYASHI Y, OISO Y, MURATA Y (2009) Prevention of neural tube defects by loss of function of inducible nitric oxide synthase in fetuses of a mouse model of streptozotocin-induced diabetes. Diabetologia, 52(5): 962-971.
THEILER K (2013) The house mouse: atlas of embryonic development. Springer Science & Business Media.
TRIPATHI V, VERMA J (2014) Different models used to induce diabetes: a comprehensive review. Int J Pharm Pharm Sci, 6(6): 29-32.
VELAZQUEZ DVO, XAVIER HS, BATISTA JEM, DE CASTRO-CHAVES C (2005) Zea mays L. extracts modify glomerular function and potassium urinary excretion in conscious rats. Phytomedicine, 12(5): 363-369.
WAHABI H, FAYED A, ESMAEIL S, MAMDOUH H, KOTB R (2017) Prevalence and complications of pregestational and gestational diabetes in Saudi women: analysis from Riyadh mother and baby cohort study (RAHMA). Biomed Res Int, 2017: 6878263.
WAHABI HA, FAYED A, ESMAEIL SA (2014) Maternal and perinatal outcomes of pregnancies complicated with pregestational and gestational diabetes mellitus in Saudi Arabia. J Diabetes Metab, 5(7): 1-5.
WANG F, REECE EA, YANG P (2015) Oxidative stress is responsible for maternal diabetes-impaired transforming growth factor beta signaling in the developing mouse heart. Am J Obstet Gynecol, 212(5): 650.e1-650.e11.
WILDE JJ, PETERSEN JR, NISWANDER L (2014) Genetic, epigenetic, and environmental contributions to neural tube closure. Ann Rev Genet, 48: 583-611.
ZHAO W, YIN Y, YU Z, LIU J, CHEN F (2012) Comparison of anti-diabetic effects of polysaccharides from corn silk on normal and hyperglycemia rats. Int J Biol Macromol, 50(4): 1133-1137.
ŽILIĆ S, JANKOVIĆ M, BASIĆ Z, VANČETOVIĆ J, MAKSIMOVIĆ V (2016) Antioxidant activity, phenolic profile, chlorophyll and mineral matter content of corn silk (Zea mays L): Comparison with medicinal herbs. J Cereal Sci, 69: 363-370.