The acute exocrine pancreatic injury was induced by L-arginine in rats and the potential prophylactic/therapeutic role of probiotics was evaluated in a histological, immunohistochemical, and molecular study. Thirty adult male rats were used and randomly divided into five groups. Group I (Control group). Group II (Probiotic group): supplemented orally with 2X108 CFU/kg Lactobacillus Acidophilus for 5 days. Group III (Acute pancreatitis, AP) group: received double intraperitoneal injections of 250mg/100g BW L-arginine with one hour apart. Group IV (Prophylactic group): before induction of AP, the rats were supplemented orally with 2X108 CFU/kg Lactobacillus Acidophilus for 5 days. Group V (Therapeutic group): after induction of AP, the rats were supplemented orally with 2X108 CFU/kg Lactobacillus Acidophilus for 5 days. Pancreatic specimens were processed for histological and biochemical studies, and assessment of deoxyribonucleic acid (DNA) fragmentation by gel electrophoresis. Serum amylase, lipase and IL-1b were determined. Also, pancreatic malondialdehyde (MDA), reduced glutathione (GSH) and myeloperoxidase (MPO) were assessed. In AP group, the histological architecture of the exocrine pancreas was distorted with acinar cell necrosis, inflammatory cellular infiltration, interstitial edema, and hemorrhage. Inducible nitric oxide synthase (iNOS) was highly expressed in the cytoplasm of acinar cells. Moreover, AP group displayed much DNA fragmentations and a significant increase of amylase, lipase, IL-b, MDA, MPO, and a decrease of GSH. Most deleterious effects of the AP were alleviated more with the prophylactic than therapeutic use of Lactobacillus Acidophilus. In conclusion: The prophylactic regimen of probiotics has a supreme beneficial effect over the therapeutic one in ameliorating the AP.
Histological evaluation of L-arginine-mediated exocrine pancreatic injury in rats and the potential implication of the probiotic (Lactobacillus Acidophilus)
Dalia A. Mandour, Asmaa M. Tolba
Department of Human Anatomy and Embryology, Faculty of Medicine, Zagazig University, Postal Code: 44519, Egypt
SUMMARY
Sign up or Login
INTRODUCTION
Acute pancreatitis (AP) is a devastating life-threatening inflammatory disease of the pancreas, and it constitutes the most common cause of hospital admission among gastrointestinal diseases (Melo et al., 2010; Mirmalek et al., 2016). Over the last few years, the incidence of AP was increased worldwide due to the high prevalence of gallstone-related disorders together with alcohol abuse, which represent two major risk factors in developing AP (Krishna et al., 2017; Esteban-Zubero et al., 2018). AP is a multifactorial disease, whose exact etiology has not been fully elucidated. However, some proposed causes are documented in the literature including trypsin reflux, a viral infection like coxsackievirus, hypercalcemia, hyperlipidemia, drugs like furosemide, thiazides, and sulfonamides or developmental anomalies particularly the pancreatic divisum (Vinklerová et al., 2010; Biradar and Veeresh, 2013). Also, the cause of the AP may be idiopathic (Muddana et al., 2009). Clinically, the presentation of AP varies from a mild self-limiting form to a severe form, necrotizing pancreatitis, which is usually encountered with systemic complications like systemic inflammatory response syndrome (SIRS) and multiple organ dysfunction syndromes (MODS), which are responsible for high AP-induced mortality (Pan et al., 2017). The overall estimated mortality rate in patients with AP is approximately 5%, which reaches 30% in patients with severe form (Shah et al., 2018). In the past decades, intense efforts have been devoted by the scientists to clarify the pathogenesis of AP. However, the exact mechanism has not been fully elucidated yet (Goodchild et al., 2019). Nevertheless, a hypothesis has argued that the pathogenesis is related to acinar cell response to the premature activation of pancreatic proenzymes, particularly trypsinogen into trypsin, which triggers a chain of activation of other pancreatic precursors that ultimately cause intracellular autodigestion of the gland (Bhatia et al., 2005; Pan et al., 2017). This process harbors many histological alterations, local inflammatory reactions, and the release of a vast array of inflammatory mediators in conjunction with free radicals in the pancreatic parenchyma. These histochemical cross talks eventually lead to acinar cell necrosis (Bhatia et al., 2005). Unfortunately, there is no specific treatment for the AP, so far and the currently available medical treatment is limited only to some supportive symptomatic drugs like analgesics, anti-inflammatories and steroids (Muddana et al., 2009; Biradar and Veeresh, 2013). These synthetic drugs were reported to be neither specific nor implemented for all patients due to the increased frequency of their complications and their high economic burden. Therefore, the search continues for novel strategies of natural compounds that are recommended based on their cost-effectiveness and pursued as prophylactic or therapeutic agents for the management of AP. In that regard, a naturally-occurring probiotic (Lactobacillus Acidophilus) was tried in this study. According to the definition adopted by Food and Agriculture Organization (FAO) and World Health Organization (WHO), probiotics are “living non- pathogenic microorganisms which when administered in adequate amounts confer a health benefit to the host”. Therefore, they have been incorporated in various fermented milk products (Zielińska and Kolożyn-Krajewska, 2018). Interestingly, probiotics involve many species of microorganisms, of which Lactobacillus acidophilus (L. acidophilus) is the most beneficial species, and it has been studied widely in animals and humans (Wang et al., 2017a). This species exerts potent anti-inflammatory, antioxidant, immune-modulatory, and bacteriostatic functions (Lutgendorff et al., 2008; Spyropoulos et al., 2011; Kechagia et al., 2013). Taking into account the potential implication of inflammatory mediators and free radicals in the pathophysiology of AP, together with the above-mentioned biological functions of the probiotics, consequently this study was settled down as a trial to explore the possible prophylactic and therapeutic role of L. acidophilus supplementation in an experimental model of AP induced by L-arginine in the adult male rats.
MATERIALS AND METHODS
Chemicals and Drugs
L-Arginine
L-arginine (a semi-essential amino acid) was provided in the form of a powder (L-arginine, Cat. No. A5006, Sigma Aldrich Company, St. Louis, MO, USA). It was dissolved in a freshly prepared normal saline (0.9% NaCl) whose pH was adjusted to 7.0 by 5.0 N HCL with a final concentration of 500 mg/ml.
Lactobacillus acidophilus (L. acidophilus)
L. acidophilus strain of probiotics was used. It was provided as tablets. Each tablet was 0.5 mg containing 108 colony-forming units (CFU) of L. acidophilus (Puritan’s Pride, INC., Holbrook, NY 11741, USA). Each tablet was dissolved in one ml of distilled water.
Experimental animals
Thirty adult male Sprague Dawley albino rats of 180-220 gm body weight (BW) were used in this study. They were obtained from the animal house of the Faculty of Medicine, Zagazig University, Egypt. The rats were left to acclimatize for one week prior to the start of the experiments. The animals were housed in clean, well-ventilated cages at a constant room temperature of 23±2°C under the natural 12-hour day/night cycles with free access to a standard commercial rodent pellets chow and water ad libitum. The rats were kept under the same environmental conditions throughout the whole study period. The experimental protocol and all experimental procedures of this study were revised and approved by the ethical guidelines established by the Institutional Animal Care and Use Committee of Zagazig University, Egypt (reference number ZU-IACUC/3/F/106/2019) in compliance with and in according to the international guidelines for the care and use of the research laboratory animals.
Experimental design
The rats were randomly divided into 5 groups, each comprising 6 rats.
Group I (Control group): The rats of this group were injected intraperitoneally (I.P) with a double dose of 1 ml normal saline with one-hour interval and left without any medication for 5 days; then they were sacrificed.
Group II (Probiotic group): The rats of this group were supplemented orally with L. acidophilus in a dose of 2X108 CFU/kg, once a day for 5 consecutive days; then they were sacrificed (Muftuoglu et al., 2006).
Group III (AP group): Induction of AP was performed by injecting the rats with double I.P doses of L-arginine with one hour apart, each of which was 250 mg/100 g BW. The animals were sacrificed 5 days after the second L-arginine injection (Pezzilli and Fantini, 2006).
Group IV (Prophylactic group): The rats were supplemented orally with L. acidophilus in a dose of 2X108 CFU/kg, daily for 5 successive days, then AP was induced as in group III and the animals were sacrificed 5 days after the second L-arginine injection.
Group V (Therapeutic group): In this group, AP was induced as in group III and after the second L-arginine injection, the rats were supplemented orally with L. acidophilus in a dose of 2X108 CFU/kg, daily for 5 successive days, after which they were sacrificed.
Induction of AP
In this study, an experimental model of AP was induced in rats via double I.P injections of L-arginine (250 mg/100g BW) with one hour apart. To confirm a successful induction of AP, two blood samples were withdrawn from the retro-orbital venous plexus of the rats; the first sample was obtained immediately before induction of AP, while the second sample was obtained 24 hours after the second injection of L-arginine. AP was biochemically insured when the serum level of amylase and lipase enzymes in the second sample was double or more than their normal basal level of the first sample (Pacheco et al., 2003).
Experimental procedures
The experimental procedures of this study were performed in the animal house of the Faculty of Medicine, Zagazig University, Egypt. At the end of the experimental schedule, the animals were subjected to overnight fasting, after which they were weighed, and then anesthetized by I.P injection of sodium pentobarbital (40 mg/kg BW). Afterward, blood samples were collected from the rats of all groups and left to be coagulated at room temperature, then centrifuged at 3000 r.p.m for 10 min. The serum was separated and collected in dry clean Eppendorf tubes, and frozen immediately at −20°C until further biochemical analysis of serum amylase, lipase, and interleukin-1beta (IL-1β).
Thereafter, the rats were sacrificed by cervical dislocation, then a midline abdominal incision was performed and tissue fixation was carried out by means of intracardiac injection of 2.5% phosphate-buffered glutaraldehyde solution (pH 7.4). Then, the whole pancreas was quickly excised outside the rats’ body, trimmed free of fat and peripancreatic tissues and blotted on filter paper, and weighed. Thereafter, the harvested pancreas was sharply divided into two halves. The half including the head was processed for light and electron microscopic examinations, while the other half was preserved in buffered saline at -80°C for later homogenization.
Pancreatic weight/body weight ratio (PW/BW ratio)
This ratio was calculated (mg/g) as a crude index of pancreatic edema (Biradar and Veeresh, 2013).
Histological study
Light microscopy (LM)
Pancreatic specimens were fixed overnight in 10% neutral buffered formalin, dehydrated in ascending grades of ethanol, cleared with xylene and embedded in paraffin wax. Paraffin sections of 5 μm thick were cut and stained with Hematoxylin and Eosin (H&E) to study the histological architecture of the exocrine pancreas (Bancroft and Layton, 2013). Finally, the stained sections were examined under a light microscope (Leica Microsystems, Schweiz, AG, Heerbrugg, CH-9435, Switzerland) and photographed using a digital camera coupled to that microscope in the Department of Anatomy, Faculty of Medicine, Zagazig University, Egypt.
Transmission electron microscopy (TEM)
Specimens from the head of the pancreas, about 1 mm3 in size, were taken, fixed in 2.5% phosphate-buffered glutaraldehyde solution (pH 7.4) for 24 hours at 4°C. Thereafter, the specimens were post-fixed in 1% osmium tetroxide at 4°C for one hour, then dehydrated in ascending grades of ethanol and finally embedded in epoxy resin. Afterward, semithin sections of about 1 μm thick were cut with an ultramicrotome (Leica Reichert, Ultracut, S, Germany), to be stained with 1% toluidine blue, and examined under a light microscope. Then, ultrathin sections of 70 nm were cut, mounted on copper grids, and double-stained with uranyl acetate and lead citrate (Bozzola and Russel, 1999). Finally, the obtained ultrathin sections were photographed using a JEOL JEM-1400 transmission electron microscope at Electron Microscopy Unit in the Research Center, Faculty of Agriculture, Cairo University, Egypt.
Immunohistochemical study
Inducible nitric oxide synthase (iNOS) enzyme expression in the pancreatic tissue was assessed by immunohistochemical staining technique. Paraffin pancreatic sections of 4 μm thick were mounted on a positively charged slide. These tissue sections were deparaffinized with xylene then rehydrated in descending grades of ethanol. Antigen retrieval was accomplished and endogenous peroxidase activity was blocked by 0.3% hydrogen peroxide (H2O2). Non-specific protein binding sites were blocked by 5% goat serum in phosphate-buffered saline (PBS) for 30 min. The sections were then incubated overnight at 4°C with primary rabbit anti-iNOS polyclonal antibody (Thermo Fisher Scientific, Massachusetts, USA) diluted 1:200 with PBS. Then, the slides were incubated with 2% biotinylated goat anti-rabbit secondary antibody in PBS (EnVisionTM/AP, K1396; Dako, Carpinteria, CA, USA) for 30 min. followed by the addition of a streptavidin-peroxidase solution. Afterward, diaminobenzidine (DAB) chromogen solution was added. Finally, the sections were counterstained with Mayer’s hematoxylin. The negative control slides were prepared following the same staining procedures, but with omitting the primary antibody. Positive iNOS immunostaining reaction was identified under the light microscope as brown coloration in the cytoplasm of the pancreatic acinar cells (Ramos-Vara et al., 2008).
Histomorphometric study
The mean optical density of iNOS immunoreaction was quantitatively assessed in five non-overlapped high-power fields (X400) of immune stained pancreatic sections from each animal of all groups. This measuring technique was performed using the image analyzer computer system (Leica Qwin plus microsystem version 3, Switzerland) at the Image Analyzing Unit of the Oral Pathology Department, Faculty of Dentistry, Cairo University, Egypt. The obtained data were represented as Mean±Standard deviation (Mean±SD) and were statistically analyzed.
Homogenization of pancreatic tissue
The buffered saline-preserved pancreatic tissue was blotted dry, weighed and homogenized 5% (w/v) in a freshly prepared ice-cold PBS (50 mM, pH 7.4) using an electronic tissue homogenizer (Model silent crusher-M; Heidolph Instruments, Donau, Germany). The resulting pancreatic homogenate was centrifuged at 4000 r.p.m for 15 min. at 4°C (Mirmalek et al., 2016). Then, the supernatant was separated and stored at -80°C in aliquots until the assessment of DNA fragmentation by gel electrophoresis and measuring the pancreatic tissue level of malondialdehyde (MDA, a marker of oxidative stress and lipid peroxidation), reduced glutathione (GSH, a marker of tissue antioxidant activity), myeloperoxidase enzyme activity (MPO, a marker of neutrophil sequestration and infiltration in pancreatic tissue).
Molecular study (Assessment of DNA fragmentation)
DNA fragmentation of the pancreatic tissue was assessed by the agarose gel electrophoresis technique adopted by Kratz and Siegfried (2010), in which DNA fragments were separated and then assessed qualitatively according to their size. Briefly, DNA was isolated from the previously prepared pancreatic homogenate of different groups, and loaded into wells at one end of agarose gel, and then an electric current was applied, DNA fragments being negatively charged; they phoresis (migrate) in lanes corresponding to their wells towards the positive electrode, where small fragments move faster than large ones through the gel. The gel-containing DNA was stained with ethidium bromide and DNA fragments were visualized as bands (each representing a group of the same sized fragments) under ultraviolet light (UV transilluminator, BIORAD, USA). Finally, DNA fragmentation was assessed based on the size of each band compared to a standard “yardstick” ladder made up of DNA fragments of known size.
Biochemical study
Serum amylase (U/L) was determined by the colorimetric method using an amylase commercial assay kit (Ultra diagnostics, Cairo, Egypt) (Ceska et al., 1969). Serum lipase (U/L) was determined by the colorimetric method using lipase commercial assay kit (Accurex diagnostics, Cairo, Egypt) (Whitaker, 1973). Serum IL-1β (pg/mL) was measured by the enzyme-linked immunosorbent assay (ELISA), following the manufacturer’s manual of instructions, using an ELISA kit (R&D Systems, Minneapolis, MN USA). The results were calculated from a standard curve and expressed as (pg/mL) (Moody et al., 2001). Pancreatic tissue MDA (nmol/g protein) was measured by thiobarbituric acid colorimetric method using MDA assay kit (Bio-diagnostic, catalog no. MD 2529, Cairo, Egypt) (Ohkawa et al., 1979). Pancreatic tissue GSH (µmol/g protein) was measured colorimetrically using GSH commercial assay kit (Bio Diagnostic, catalog no. GR 2511; Cairo, Egypt) (Beutler et al., 1963). Pancreatic tissue MPO activity (U/g protein) was assessed colorimetrically according to the manufacturer’s instructions, using MPO commercial assay kit (Nanjing Jian cheng Corp., China) (Bradley et al., 1982).
Statistical analysis
The morphometric and biochemical studies were statistically analyzed using the statistical package of Social Science Program, Version 19 for Windows (SPSS Inc., Chicago, Illinois, USA). The data were expressed as Mean±SD. Statistical significance was calculated by using one-way ANOVA, followed by post hoc Tukey’s test for multiple comparisons. P-value ≤0.05 was considered statistically significant (Petrie and Sabin, 2005).
RESULTS
During the experiment, no mortality was recorded among the rats of all groups.
Light microscopic
Examination of (H&E) stained pancreatic sections of the control and probiotic groups revealed a normal histological architecture of the pancreas, which appeared to be divided into lobules by thin interlobular connective tissue septa containing interlobular ducts. The lobules contained closely packed exocrine acini, which appeared rounded or triangular in cross-section with basal basophilia and apical eosinophilia. The acini have central lumen contained centroacinar cells. Among the acini, the endocrine islets of Langerhans were embedded and appeared as clusters of pale staining cells intermingled with blood sinusoids with no apparent capsule (Figs. 1a, 1b). Moreover, toluidine blue-stained sections of the control and probiotic groups revealed pancreatic acini lined with pyramidal-shaped cells that had basal rounded vesicular nuclei with many apical, densely-stained, secretory zymogen granules (ZG). The acinar cell boundaries were regular and well defined, and the acini were separated from each other by thin inter-acinar connective tissue septa (Figs. 2a, 2b).
Examination of H&E-stained sections of the AP group demonstrated a massive distortion of the histological architecture of the pancreatic acini, with focal areas of acinar cell necrosis in the form of multiple vacuoles in diffuse eosinophilic cytoplasm, giving the tissue a moth-eaten appearance with pyknotic, karyorrhectic, and karyolytic nuclei (Fig. 1c). Also, there were wide interlobular septa containing dilated, congested blood vessels (BVs) with inflammatory cellular infiltrate and extravasation of inflammatory exudate entangled with red blood cells. The distorted acini were separated by wide inter-acinar spaces with microscopic foci of interstitial hemorrhage from destructed blood vessels (Fig. 1d). Furthermore, toluidine blue sections of the AP group demonstrated loss of acinar cell boundaries, and the cytoplasm contained few or depleted ZG. The nuclei appeared small irregular, pyknotic and karyolytic. Some acini were anucleated (Fig. 2c).
Examination of H&E sections of the prophylactic group showed preserved pancreatic architecture, where the pancreatic lobules revealed some intact acini, while some appeared distorted with wide inter-acinar and interlobular spaces (Fig.1e). Moreover, toluidine blue sections of this group revealed some preserved acinar cells with basal vesicular nuclei and many secretory ZG. However, some acini showed irregular nuclei (Fig. 2d).
Examination of H&E sections of the therapeutic group revealed somewhat comparable changes to that of the AP group, where some acini showed necrotic changes in the form of vacuolations in diffuse eosinophilic cytoplasm with pyknotic, karyolitic, and karyorrhectic nuclei (Fig. 1f). Additionally, toluidine blue of this group exhibited loss of the cell boundaries of some acini with an intermingling of their ZG. Most of the acini showed irregular, pyknotic and karyolytic nuclei (Fig. 2e).
Transmission electron microscopic
TEM examination of the pancreatic acinar cells of the control and probiotic groups displayed nearly the same ultrastructural picture of the normal acinar cells, having rounded euchromatic nuclei with dispersed chromatin and prominent nucleoli. The cytoplasm contained a heavy basal arrangement of the rough endoplasmic reticulum (rER) with parallel-arranged cisternae yielding a lamellar profile, surrounding the nucleus. Mitochondria of normal shape and size were interspersed within rER. The apical part of the acinar cells was occupied by numerous ZG that appeared homogenous electron-dense (Figs. 3a, 3b, and 3c).
TEM examination of pancreatic acinar cells of the AP group showed small, irregular, fragmented and heterochromatic nuclei with dark peripheral condensed chromatin. Most acinar cells showed ZG of variable size and electron-density. Also, dilated fragmented cisternae of rER and swollen mitochondria were observed. Some acinar cells had rarified electron-lucent areas of cytoplasm that were nearly devoid of organelles. Congested BVs with perivascular inflammatory exudates were observed (Figs. 3d, 3e, and 3f).
Ultrathin sections of the acinar cells of the prophylactic group revealed a cytoplasm with less electron-dense ZG, apparently normal mitochondria, and heterochromatic nuclei surrounded by some regularly arranged cisternae of rER with other dilated and disarranged ones (Fig. 4a). Ultrathin sections of the acinar cells of the therapeutic group revealed irregular small heterochromatic nuclei, dilated fragmented cisternae of rER, swollen mitochondria, ZG of variable size, and electron density (Figs. 4b, 4c).
Immunohistochemical results
Examination of the iNOS immunohistochemical-stained sections from the control and probiotic groups appeared nearly similar, where a negative immunostaining reaction for iNOS in the cytoplasm of the normal acini with a weak immunoreaction in the cytoplasm of islets of Langerhans cells in the form of faint brown staining was noticed (Figs. 5a, 5b). AP group showed a strong positive immunostaining reaction for iNOS with a high intense brown coloration of the cytoplasm of the acinar cells and the wall of BVs compared with the control and probiotic groups (Fig. 5c).
However, the prophylactic group showed a mild immunostaining reaction for iNOS in the cytoplasm of both acinar cells and the islets of Langerhans with less intense brown coloration compared with that of the AP group, but more intense coloration when compared with the control and probiotic groups (Fig. 5d). The therapeutic group showed a moderate immunostaining reaction for iNOS in the cytoplasm of both acinar cells and islets of Langerhans, with less intense brown coloration than AP but more intense than the control and probiotic groups (Fig. 5e).
Histomorphometric results
The mean value of iNOS in the cytoplasm of the acinar cells of the AP group was 71.28±8.32, which was statistically significantly higher (P<0.0001) compared with those of the control group (7.57±1.19) and probiotic group (7.24±1.30). The mean values of iNOS in both prophylactic and therapeutic groups were 44.66±2.14 and 59.18±4.18 respectively, which were statistically significantly lower (P<0.0001 and P<0.001), compared with that of the AP group (Fig. 6).
DNA fragmentation results
The results of the gel electrophoresis displayed five DNA bands in lanes corresponding to their wells, which were regularly arranged from I to V, corresponding to the experimental groups of this study. The wells I (control group) and II (probiotic group) showed clear sharp normal DNA bands. Well III (AP group) revealed a swarming of the DNA band with many fragmentations. Well IV (prophylactic group) showed a DNA band with minor fragmentation. Well V (therapeutic group) showed a comparable DNA band to that of the AP group, but with lesser fragmentation (Fig. 7).
PW/BW ratio results
The mean value of the PW/BW ratio in the AP group was 9.51±0.67, which was statistically significantly higher (P<0.0001) compared with those of the control group (6.08±1.02) and the probiotic group (5.91±0.85). The mean values of the PW/BW ratio in both prophylactic and therapeutic groups were 7.60±0.55 and 7.95±1.20 respectively, which were statistically significantly lower (P<0.008 and P<0.04) compared with that of the AP group (Fig. 8).
Biochemical results
The results of serum amylase, lipase, and IL-1β were depicted in the Table 1. Statistical comparison between the control and the probiotic groups revealed insignificant change (P>0.05) regarding all the measured biochemical parameters. In the AP group, the mean serum amylase, lipase, and IL-1β levels were significantly higher (P=0.0001) when compared with the corresponding values of the control group. In the prophylactic group, the serum level of these three biochemical parameters decreased significantly (P=0.0001, P=0.002, and P=0.004), respectively when compared with the AP group. In the therapeutic group, the serum level of the above-mentioned three parameters showed insignificant differences (P=0.47, P=0.74, and P=0.12), respectively when compared with the AP group. In the therapeutic group, the mean serum amylase and lipase were significantly higher (P=0.015 and P=0.041), while the mean serum IL1B was insignificantly changed (P=0.535) when compared with the corresponding values of the prophylactic group.
The results of the pancreatic tissue level of MDA, GSH, and MPO were depicted in the Table 2. In the AP group, the mean pancreatic tissue MDA was significantly higher (P=0.0001) when compared with the corresponding values of the control group. In the prophylactic and therapeutic groups, the MDA level decreased significantly (P=0.0001 and P=0.024), respectively when compared with the AP group. The mean pancreatic tissue level of GSH in the AP group was significantly lower (P=0.0001) than those of the control group. In the prophylactic and therapeutic groups, GSH levels displayed insignificant change (P=0.48 and P=0.78), respectively compared with the AP group. In the AP group, the mean pancreatic tissue MPO was significantly higher (P=0.0001) when compared with the corresponding value of the control group. In the prophylactic group, the MPO level decreased significantly (P=0.0001) when compared with the AP group, while in the therapeutic group, MPO displayed insignificant change (P=0.27) when compared with the AP group. In the therapeutic group, the mean serum MDA and MPO were significantly higher (P=0.032 and P=0.001), while the mean serum GSH was insignificantly changed (P=0.98) when compared with the corresponding values of the prophylactic group.
DISCUSSION
Acute pancreatitis (AP) is a major inflammatory disease of the pancreas that, to date, has neither satisfactory settled underlying pathogenesis nor conventional successful therapy. So far it is thus usually associated with a high rate of morbidity and mortality (Esteban-Zubero et al., 2018). In this study, an experimental model of AP was produced by I.P injection of a high dose of L-arginine: this amino acid was reported to produce selective dose-dependent necrosis of the exocrine part of the pancreas without a major morphological change in the endocrine part or other organs (Su et al. 2006 and Dawra; Saluja, 2012).
This model was chosen because it is an easy, highly reproducible, and a non-invasive model. Besides, it produces histological and biochemical alterations in the acinar cells resembling those of acute necrotizing pancreatitis in humans (Su et al. 2006). In a trial to ameliorate such histochemical changes during AP, L.acidophilius species of the probiotics were tried in this study. The results of the present study revealed a marked distortion of the histological architecture of the pancreatic acini of the AP group and focal areas of acinar cell necrosis associated with interstitial edema, microscopic foci of hemorrhage, and inflammatory cellular infiltration. These findings were in line with Melo et al. (2010); Chen et al. (2012), and Wang et al. (2017b), who reported that a large dose of L-arginine in different experimental animals resulted in pancreatic histopathological changes nearly similar to those observed in this study.
The mechanism by which L-arginine produced the above-mentioned pancreatic histological alterations has been attributed partly to the excessive production of nitric oxide (NO) in the pancreatic tissue. NO is a potential free radical, and is one of the most important inflammatory mediators generated from L-arginine in a reaction catalyzed by a family of three NO synthase (NOS) isoforms, including neuronal NOS (nNOS), endothelial NOS (eNOS), and inducible NOS (iNOS) (Takacs et al., 2002). On the contrary to nNOS and eNOS , which are a normal cellular constituent and form a small amount of NO, iNOS is not a cellular constituent, but it is cytokine-inducible and forms a large amount of NO in a sustained and uncontrolled manner in cases of inflammatory condition of a wide variety of tissues, particularly the pancreas (Tanjoh et al., 2007; Förstermann and Sessa, 2011).
The sustained overproduction of NO imparts a direct toxic effect on the pancreatic acinar cells, and mediates diverse inflammatory responses like interstitial edema, and inflammatory cellular infiltration (Tanjoh et al., 2007). Additionally, much evidence implicated that excessive NO reacts with superoxide anion radicals (O2--), resulting in the formation of peroxynitrite anions (ONOO¯), which are highly reactive nitrogen species (RNS) and represent powerful oxidants and cytotoxic agents that have been incriminated indirectly in the pathogenesis of acinar cell injury (Bhatia et al., 2005; Dawra and Saluja, 2012). In the present study, the increased iNOS activity in the pancreatic tissue of the AP group was evident, where there were strong positive immunohistochemical staining reactions and an increase in the mean morphometric optical density of antibody-iNOS reaction inside the cytoplasm of the acinar cells and the wall of microvasculature, compared with the negative reaction seen in the control group. However, the latter group showed a weak staining reaction in the cytoplasm of the islet cells.
These results were explained by Qader et al. (2003), who reported that iNOS is not a normal constituent in the exocrine compartment of the pancreas, but it is weakly expressed in the islets of Langerhans. Apparently in this study, there was a decrease of iNOS expression upon probiotic supplementation, particularly in the prophylactic group of rats that might mitigate NO production. This finding could explain how the pancreatic histopathological changes were indirectly ameliorated by these natural non-pathogenic microorganisms. The iNOS was reported to be highly induced by tissue inflammatory cytokines and endotoxins (Al-Mufti et al., 1998). Therefore, the downregulation of iNOS elicited by the currently used L. acidophilus could be due to their anti-inflammatory effects (Kechagia et al., 2013) and their ability to inhibit bacterial endotoxins (Markowiak and Śliżewska, 2017). Determinately, not only NO and RNS, but also reactive oxygen species (ROS) produced from the injured acinar cells are reported to be strongly implicated in the pathogenesis of L-arginine-induced AP. It was cited that these ROS, in a positive feedback manner, cause more acinar cell injury via oxidative denaturation of the proteins of acinar cell membranes, leading to loss of their structural integrity, with subsequent extracellular leakage of pancreatic protease enzymes that ultimately lead to necrosis of the gland (Esrefoglu, 2012). Also, ROS cause denaturation of cytoplasmic proteins, cellular organelles, and the cytoskeleton yielding more necrosis (Urunuela et al., 2002).
Correspondingly, in this study, upon examining LM and TEM pancreatic sections in the AP group, a full-blown picture of acinar cell necrosis was observed in the form of loss of acinar cell boundaries, vacuolated rarified cytoplasm, and even acinar cell loss giving the tissue a moth-eaten appearance. Also, pyknotic, karyolitic, and karyorrhectic nuclei were encountered. The later changes in the nuclei were reported to be mostly due to ROS-induced strand breaks, abnormal cross-linking, and fragmentation of DNA (Meher et al., 2015). Furthermore, ROS lead to peroxidation of polyunsaturated fatty acids of the acinar cell membranes, resulting in the formation of toxic byproducts metabolites such as MDA (Özkan et al., 2012). In that regard, in the present study, pancreatic MDA was measured, and its level was significantly high in the AP group. Likewise, Mirmalek et al. (2016) have found that the MDA level increased significantly in rats with L-arginine-induced AP. On the other hand, in this study, L. acidophilus supplementation prior to induction of AP resulted in a stronger reduction of MDA than with the post induction regimen of the therapeutic group. This result might emphasize the antioxidant effect of probiotics (Spyropoulos et al., 2011).
In this study, redox imbalance was evident in the AP group, where not only the pancreatic MDA was increased, but also GSH, the main intracellular non-enzymatic antioxidant, was significantly decreased compared to the control group. In support of our findings, Pérez et al. (2015) have documented an early depletion of GSH in pancreatic tissue in AP. This depletion is presumably due to overconsumption of GSH in catalyzing premature activation of pancreatic proenzymes into active enzymes (Lüthen et al., 1998). Nevertheless, in this study, there was a mild increase in GSH that did not reach a significant level in the prophylactic and therapeutic groups compared with the AP group. This implied mostly that the chosen dose of L. acidophilus had a moderate capability in reducing oxidative stress (Lutgendorff et al., 2008).
In this study, one of the main histological findings in the AP group was the perivascular inflammatory cellular infiltration in the pancreatic tissue. Pathologically, in acute inflammation, the leucocytes, in particular the neutrophils, are known to be recruited and activated within hours to the inflammatory sites by the chemotactic effect of the cytokines, released from both injured pancreatic tissue and the resident pancreatic macrophages (Bhatia et al., 2005; Cosen-Binker and Gaisano, 2007). Once activated, the neutrophils start their strong phagocytic activity and degranulate their granules, particularly the primary (azurophilic) granules that contain an ample amount of myeloperoxidase (MPO) enzyme (Selders et al., 2017).
Therefore, biochemical assessment of the tissue activity of MPO is used as an indirect biomarker of the neutrophil infiltration and as an index of acute inflammation of different tissues (Biradar and Veeresh, 2013). Correspondingly, in this study, neutrophils’ trafficking to the inflamed pancreatic tissues in the AP group was confirmed biochemically by the elevated pancreatic tissue level of MPO. Obviously in the present study, upon histological examination of the pancreatic tissue of the AP group, the pancreatic acini were separated by wide inter-acinar and interlobular spaces and inflammatory exudate. Widening of these spaces was secondary to the encountered necrosis of the pancreatic acini (Melo et al, 2010; Chen et al., 2012). Whilst, the exudate may reflect the presence of interstitial pancreatic edema (Wang et al. 2017b). The later histological finding was broadly supported by what was obtained in this work on measuring the PW/BW ratio, which represents a rough index in evaluating the degree of pancreatic edema. The cause of such edema is the increase of microvascular permeability, which is caused presumably by the potent vasodilator effect of NO and by the proinflammatory cytokines released from the injured pancreatic acini (Takacs et al., 2002). Also, in this study, microscopic foci of interstitial hemorrhage were observed in the histological sections of the AP group. The cause of such hemorrhage is the proinflammatory cytokines that cause vascular endothelial injury with extravasation of blood in the interstitium (Wang et al., 2017b).
Keeping in view the above-mentioned deleterious roles of the cytokines in the pathogenesis of the AP, consequently in the present study, the serum level of the proinflammatory cytokine IL-1β was measured and its level was significantly higher in the AP group compared with the control one. This was consistent with Özkan et al. (2012) and Mirmalek et al. (2016), who found that serum IL-1β level increased in different rat models of AP. On the other side, IL-1β decreased significantly more in the prophylactic than in the therapeutic group, denoting a more beneficial anti-inflammatory effect of L. acidophilus pretreatment.
In light of the pathophysiology of AP, emerging evidence has shown that many cytokines rather than IL-1β such as tumor necrosis factor alpha (TNF-α) and IL-6 are produced during AP from the injured acini, macrophages, and recruited leucocytes (Cosen-Binker and Gaisano, 2007). Uncontrolled release of these cytokines induces premature activation of the pancreatic proenzymes (zymogens) (Tsang et al., 2016). Also, ROS causes denaturation of the membrane proteins of lysosomes and ZG yielding, their co-localization forming cytoplasmic vacuoles in which the lysosomal hydrolase enzymes such as cathepsin-B causes premature activation of the pancreatic zymogens (Waterford et al., 2005). Moreover, ROS causes thiol oxidation of cysteine residues of ryanodine receptors (RyR) and inositol trisphosphate receptors (IP3R) of calcium (Ca2+) channels on the smooth endoplasmic reticulum (sER), resulting in hastening the activity of these channels with subsequent active efflux of Ca2+ into the cytosol. This rise of the intracellular Ca2+ induces premature activation of pancreatic zymogens (Husain et al., 2005). In this premature activation process, trypsinogen, in particular, is activated into trypsin, which is the most potent pancreatic proteases in the initiation of the AP. Once activated, trypsin is capable of converting other zymogen forms of pancreatic enzymes to their active forms (Tsang et al., 2016).
For instance, activation of chymotrypsin, carboxypeptidase, and aminopeptidases cause denaturation of cytoplasmic proteins; activation of phospholipases disrupts the membrane phospholipids; activation of endonucleases causes DNA chromatin damage, and activation of elastase disrupts the elastic fibers in the wall of BVs, causing interstitial hemorrhage (Kumar et al., 2018).These inappropriately activated pancreatic enzymes elicit autodigestion (autolysis) of the gland (Bhatia et al., 2005 and Wang et al. 2017b). This autodigestion process is an overwhelming necrotic process, particularly of the exocrine pancreas, in which the cytoplasm showed diffuse eosinophilia, which is attributable partly to increased binding of eosin stain to the denatured cytoplasmic proteins, and partly to the loss of basal basophilia secondary to denatured ribonucleoproteins in rER.
On the contrary, in normal acinar cells, eosinophilia is restricted only to the apical part due to abundant ZG (Kumar et al., 2018). Interestingly, premature activation of pancreatic enzymes with subsequent autodigestion of the gland represents hallmarks in the pathogenesis of experimental AP (Pan et al., 2017). In the present study, in addition to the above-mentioned histological findings, the development of AP was confirmed biochemically by a significant increase in the serum amylase and lipase enzyme levels that decreased with L. acidophilus administration. In agreement with these results, Melo et al. (2010) and Chen et al. (2012) have found that the serum amylase and lipase increased significantly in animal models of AP. The cause beyond such rise of these two enzymes could be attributed to the proteolysis of ZG and cytoskeleton proteins of the acinar cells, with the subsequent escape of these enzymes locally into the pancreatic interstitium, and thereafter into the bloodstream (Urunuela et al., 2002 and Tsang et al., 2016).
Notably in this study, the prophylactic regimen of L. acidophilus yielding a more beneficial effect over the therapeutic one in ameliorating most of the histological and biochemical results encountered with AP. This beneficial protective role of L. acidophilus could be due to its ability in preventing secondary bacterial infection of the necrotic pancreatic tissue which is a pathological hallmark during AP (Pan et al., 2017). This secondary bacterial infection is presumably due to bacterial translocation which is a process in which intestinal microbiota crosses a dysfunctional intestinal mucosal barrier and reaches the necrotic tissue of the pancreas via systemic circulation (Guarner and Malagelada, 2003) and via mesenteric lymphatics (Mittal et al., 2009).
The prevention of secondary bacterial infection was reflected upon MPO that significantly decreased in the pancreatic tissue of the prophylactic group in comparison with that of the AP group in this study. It was reported that pre-treatment with probiotics reduced bacterial translocation through releasing many antimicrobial substances like defensins and bacteriocins (Penner et al., 2005) and exerting a potent immune-modulatory effect (Pan et al., 2017). Also, they strengthen the intestinal mucosal barrier by increasing mucosal IgA, enhance tight junction protein expression, prevent epithelial cell apoptosis, and release cytoprotective molecules such as heat shock protein (Madsen 2012). This prophylactic dogma of probiotics in maintaining gut integrity was documented in the literature, in particular with L. acidophilus species (Wang et al., 2017a). In vitro, these species were found to increase mucin secretion from human intestinal epithelial cells, which block pathogenic E. coli adherence and invasion (Mack et al., 2003). In addition, L. acidophilus produces lactic, acetic, and propionic acids, which lower the intestinal pH, thus suppressing the growth of various pathogenic bacteria and keep the balance of the gut flora (Doron and Gorbach, 2006). Based upon their above-mentioned roles, probiotics have been used in the management of infectious and antibiotic-associated diarrhea, Clostridium difficile infections, lactose intolerance, inflammatory bowel diseases, and necrotizing enterocolitis (Knight and Girling, 2003).
Conclusion
The findings of this study have shown that the prophylactic regimen of L. acidophilus has a supreme beneficial effect over the therapeutic one in ameliorating some histopathological changes of the pancreatic tissue, and in attenuating the markers of oxidative stress and inflammation in an experimental model of the AP. This crucial prophylactic effect is presumably due to the antioxidant, anti-inflammatory, and immune-modulatory effects of L. acidophilus in addition to their role in preventing secondary bacterial infection of the injured pancreatic tissue. These finding provided a basis for future clinical studies that may constitute a novel strategy in the prophylaxis against the AP in humans.
ACKNOWLEDGEMENTS
The authors are grateful to the team of Zagazig Scientific and Medical Research Center (ZSMRC) for helping the authors in accomplishing the experiments.
Author contributions: All authors contributed to the study conception and design. Material preparation, data collection, and analysis were performed by [Dalia A. Mandour]. The first draft of the manuscript was written by [Asmaa M. Tolba] and all authors commented on previous versions of the manuscript. All authors read and approved the final manuscript.
Related articles






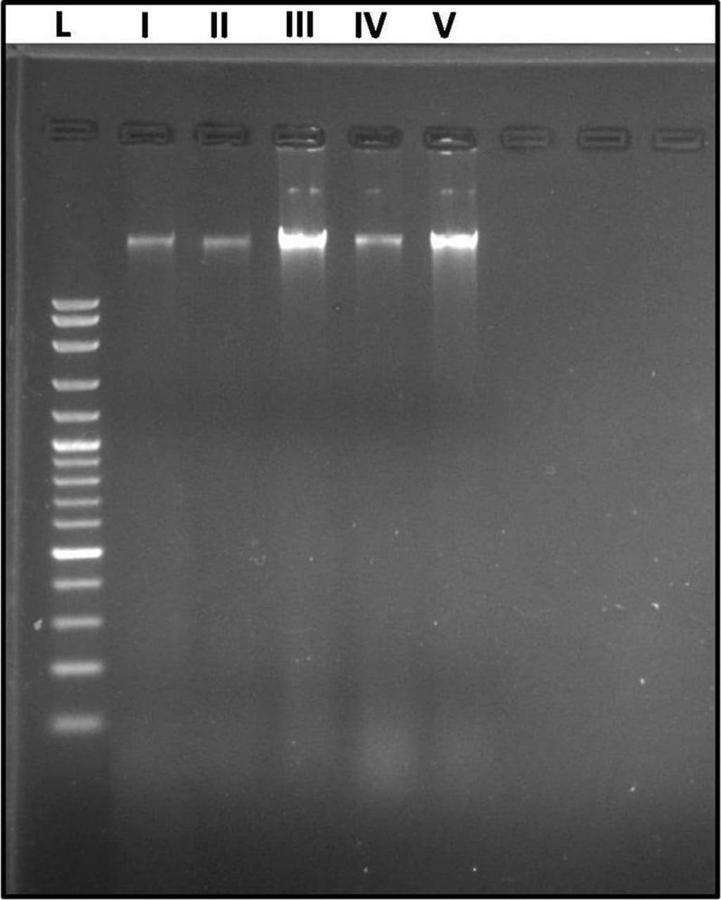


Al-MUFTI RA, WILLIAMSON RCN, MATHIE RT (1998) Increased nitric oxide activity in a rat model of acute pancreatitis. Gut, 43 (4): 564-570.
BANCROFT JD, LAYTON C (2013) The hematoxylin and eosin. In: Suvarna SK, Layton C, Bancroft JD (eds). Theory Practice of histological techniques, 7th ed. Churchill Livingstone, Elsevier, Philadelphia, USA, pp 179-220.
BEUTLER E, DURON O, KELLEY BM (1963) Improved method for the determination of blood glutathione. J Lab Clin Med, 6: 882-888.
BHATIA M, WONG FL, CAO Y, LAU HY, HUANG J, PUNEET P, CHEVALI L (2005) Pathophysiology of acute pancreatitis. Pancreatology, 5(2-3): 132-144.
BIRADAR S, VEERESH B (2013) Protective effect of lawsone on L-Arginine induced acute pancreatitis in rats. Indian J Exp Biol, 51(3): 256-261.
BOZZOLA J, RUSSEL L (1999) Electron microscopy: Principles and techniques for biologists. 2nd ed. Sudbury MA, Jones and Bartlett Publishers, Toronto, Canada, pp 17-48.
BRADLEY PP, PRIEBAT DA, CHRISTENSEN RD, ROTHSTEIN G (1982) Measurement of cutaneous inflammation: Estimation of neutrophil content with an enzyme marker. J Invest Dermatol, 78: 206-209.
CESKA M, BIRATH K, BROWN B (1969) A new and rapid method for the clinical determination of alpha-amylase activities in human serum and urine. Optimal conditions. Clin Chim Acta, 26: 437-744.
CHEN J, CAI QP, SHEN PJ, YAN RL, WANG CM, YANG DJ, FU H-B, CHEN X-Y (2012) Netrin-1 protects against L-Arginine-induced acute pancreatitis in mice. PLoS One, 7(9): e46201.
COSEN-BINKER LI, GAISANO HY (2007) Recent insights into the cellular mechanisms of acute pancreatitis. Can J Gastroenterol, 21(1): 19-24.
DAWRA R, SALUJA AK (2012) L-arginine-induced experimental acute pancreatitis. Pancreapedia Exocrine Pancreas Knowledge Base, 1: 1-6.
DORON S, GORBACH SL (2006) Probiotics: Their role in the treatment and prevention of disease. Expert Rev Anti Infect Ther, 4(2): 261-275.
ESREFOGLU M (2012) Experimental and clinical evidence of antioxidant therapy in acute pancreatitis. World J Gastroenterol, 18(39): 5533-5541.
ESTEBAN-ZUBERO E, CABRERA-FALCÓN E, RIBAGORDA-TEJEDOR S, ALATORRE-JIMÉNEZ MA, LÓPEZ-GARCÍA CA, MARÍN-MEDINA A, VILLEDA-GONZÁLEZ R, ALONSO BARRAGÁN SA, GÓMEZ-RAMOS JJ (2018) Current trends in management of acute pancreatitis. Ann Gastroenterol Dig Syst, 2(1006): 1-7.
FORSTERMANN U, SESSA WC (2011) Nitric oxide synthases: regulation and function. Eur Heart J, 33(7): 829-837.
GOODCHILD G, CHOUHAN M, JOHNSON GJ (2019) Practical guide to the management of acute pancreatitis. Frontline Gastroenterol, 10(3): 292-299.
GUARNER F, MALAGELADA JR (2003) Gut flora in health and disease. Lancet, 361(9356): 512-519.
HUSAIN SZ, PRASAD P, GRANT WM, KOLODECIK TR, NATHANSON MH, GORELICK FS (2005) The ryanodine receptor mediates early zymogen activation in pancreatitis. Proc Natl Acad Sci USA, 102(40): 14386-14391.
KECHAGIA M, BASOULIS D, KONSTANTOPOULOU S, DIMITRIADI D, GYFTOPOULOU K, SKARMOUTSOU N, FAKIRI EM (2013) Health benefits of probiotics: A review. ISRN Nutr, 2013: 481651.
KNIGHT DJW, GIRLING KJ (2003) Gut flora in health and disease. Lancet, 361(9371): 1831.
KRATZ RF, SIEGFRIED DR (2010) Using gel electrophoresis to separate molecules. In: Biology for dummies. 2nd ed. Hoboken, NJ: John Wiley and Sons, USA, pp 132-133.
KRISHNA SG, KAMBOJ AK, HART PA, HINTON A, CONWELL DL (2017) The changing epidemiology of acute pancreatitis hospitalizations: a decade of trends and the impact of chronic pancreatitis. Pancreas, 46(4): 482-488.
KUMAR V, ABBAS AK, ASTER JC (2018) Robbins Basic Pathology. 10th ed. Elsevier Philadelphia, Pennsylvania, USA, pp 680-689.
LUTGENDORFF F, TRULSSON LM, VAN MINNEN LP, RIJKERS GT, TIMMERMAN HM, FRANZEN LE, GOOSZEN HG, AKKERMANS LMA, SÖDERHOLM JD, SANDSTRÖM PA (2008) Probiotics enhance pancreatic glutathione biosynthesis and reduce oxidative stress in experimental acute pancreatitis. Am J Physiol Gastrointest Liver Physiol, 295(5): G1111-G1121.
LUTHEN R, GRENDELL JH, NIEDERAUC, HAUSSINGER D (1998) Trypsinogen activation and glutathione content are linked to pancreatic injury in models of biliary acute pancreatitis. Int J Pancreatol, 24(3): 193-202.
MACK DR, AHRNE S, HYDE L, WEI S, HOLLINGSWORTH MA (2003) Extracellular MUC3 mucin secretion follows adherence of Lactobacillus strains to intestinal epithelial cells in vitro. Gut, 52(6): 827-833.
MADSEN KL (2012) Enhancement of epithelial barrier function by probiotics. J Epith Biol Pharmacol, 5(1): 55-59.
MARKOWIAK P, ŚLIZEWSKA K (2017) Effects of probiotics, prebiotics, and synbiotics on human health. Nutrients, 9(9): 1021-1050.
MEHER S, RATH S, SHARMA R, ROUT B, MISHRA TS, SASMAL PK, SINHA MK (2015) Pathophysiology of oxidative stress and antioxidant therapy in acute pancreatitis. J Mol Biomark Diagn, 6(6): 1-7.
MELO CM, CARVALHO KMMB, DE SOUSA NEVES JC, MORAIS TC, RAO VS, SANTOS FA, DE-CASTRO BRITO GA, CHAVES M-H (2010) α, β-amyrin, a natural triterpenoid ameliorates L-arginine-induced acute pancreatitis in rats. World J Gastroenterol, 16(34): 4272-4280.
MIRMALEK SA, GHOLAMREZAEI BA, YAVARI H, KARDEH B, PARSA Y, SALIMI-TABATABAEE SA, JANGHOLI E (2016) Antioxidant and anti-inflammatory effects of coenzyme Q10 on L-arginine-induced acute pancreatitis in rat. Oxi Med Cell Long, 2016: 5818479.
MITTAL A, PHILLIPS AR, MIDDLEDITCH M, RUGGIERO K, LOVEDAY B, DELAHUNT B, COOPER GJS, WINDSOR JA (2009) The proteome of mesenteric lymph during acute pancreatitis and implications for treatment. JOP, 10(2): 130-142.
MOODY MD, ARSDELL SV, MURPHY KP, ORENCOLE SF, BURNS C (2001) Array-based ELISAs for high-throughput analysis of human cytokines. Biotech, 31: 186-194.
MUDDANA V, WHITCOMB DC, PAPACHRISTOU G (2009) Current management and novel insights in acute pancreatitis. Expert Rev Gastroenterol Hepatol, 3(4): 435-444.
MUFTUOGLU MAT, ISIKGOR S, TOSUN S, SAGLAM A (2006) Effects of probiotics on the severity of experimental acute pancreatitis. Eur J Clin Nutr, 60(4): 464-468.
OHKAWA H, OHISHI W, YAGAI K (1979) Assay for lipid peroxides in animal tissues by thiobarbituric acid reaction. Biochem, 95: 351-358.
ÖZKAN E, AKYUZk C, DULUNDU E, TOPALOGLU Ü, ŞEHIRLI AÖ, ERCAN F, SENER G (2012) Protective effects of lycopene on cerulein-induced experimental acute pancreatitis in rats. J Surg Res, 176(1): 232-238.
PACHECO RC, NISHIOKA SA, DE OLIVEIRA LC (2003) Validity of serum amylase and lipase in the differential diagnosis between acute/acutized chronic pancreatitis and other causes of acute abdominal pain. Arq Gastroenterol, 40(4): 233-238.
PAN LL, LI J, SHAMOON M, BHATIA M, SUN J (2017) Recent advances on nutrition in treatment of acute pancreatitis. Front Immunol, 8: 1-10.
PENNER R, FEDORAK RN, MADSEN KL (2005) Probiotics and nutraceuticals: non-medicinal treatments of gastrointestinal diseases. Curr Opin Pharmacol, 5(6): 596-603.
PEREZ S, PEREDA J, SABATER L, SASTERJ (2015) Redox signaling in acute pancreatitis. Redox Biol, 5: 1-14.
PETRIE A, SABIN C (2005) In: Sugden M, Moore K (eds). Medical statistics at a glance, 2nd ed. Blackwell Publishing Ltd. USA, pp 55-56.
PEZZILLI R, FANTINI L (2006). Probiotics and severe acute pancreatitis. J Pancreas (JOP), 7(1): 92-93.
QADER SS, EKELUND M, ANDERSSON R, OBERMULLER S, SALEHI A (2003) Acute pancreatitis, expression of inducible nitric oxide synthase and defective insulin secretion. Cell Tissue Res, 313(3): 271-279.
RAMOS-VARA JA, KIUPEL M, BASZLER T, BLIVEN L, BRODERSEN B, CHELACK B, CZUB S, DEL PIERO F, DIAL S, EHRHART EJ, GRAHAM T, MANNING L, PAULSEN D, VALLI VE, WEST K (2008) Suggested guidelines for immunohistochemical techniques in veterinary diagnostic laboratories. J Vet Diagn Invest, 20: 393-413.
SELDERS GS, FETZ AE, RADIC MZ, BOWLIN GL (2017) An overview of the role of neutrophils in innate immunity, inflammation and host-biomaterial integration. Regen Biomater, 4(1): 55-68.
SHAH AP, MOURAD MM, BRAMHALL SR (2018) Acute pancreatitis: current perspectives on diagnosis and management. J Inflamm Res, 11: 77-85.
SPYROPOULOS BG, MISIAKOS EP, FOTIADIS C, STOIDIS CN (2011) Antioxidant properties of probiotics and their protective effects in the pathogenesis of radiation-induced enteritis and colitis. Dig Dis Sci, 56(2): 285-294.
SU KH, CUTHBERTSON C, CHRISTOPHI C (2006) Review of experimental animal models of acute pancreatitis. HPB, 8(4): 264-286.
TAKACS T, CZAKO L, MORSCHL E, LASZLO F, TISZLAVICZ L, RAKONCZAY ZJR, LONOVICS J (2002) The role of nitric oxide in edema formation in L-arginine-induced acute pancreatitis. Pancreas, 25 (3): 277-282.
TANJOH K, TOMITA R, IZUMI T, KINOSHITA K, KAWAHARA Y, MORIYA T, UTAGAWA A (2007) The expression of the inducible nitric oxide synthase messenger RNA on monocytes in severe acute pancreatitis. Hepatogastroenterology, 54(75): 927-931.
TSANG SW, GUAN YF, WANG J, BIAN ZX, ZHANG HJ (2016) Inhibition of pancreatic oxidative damage by stilbene derivative dihydro-resveratrol: implication for treatment of acute pancreatitis. Sci Rep, 6: 22859-22873.
URUNUELA A, SEVILLANO S, De LA MANOAM, MANSO MA, ORFAO A, de DIOS I (2002) Time-course of oxygen free radical production in acinar cells during acute pancreatitis induced by pancreatic duct obstruction. Biochim Biophys Acta, 1588(2): 159-164.
VINKLEROVA I, PROCHAZKA M, PROCHAZKA V, URBANEK K (2010) Incidence, severity, and etiology of drug-induced acute pancreatitis. Dig Dis Sci, 55(10): 2977-2981.
WANG Y, WU Y, WANG Y, XU H, MEI X, YU D, WANG Y, LI W (2017a) Antioxidant properties of probiotic bacteria. Nutrients, 9(5): 521-535.
WANG N, ZHANG F, YANG L, ZOU J, WANG H, LIU K, WANG K (2017b) Resveratrol protects against L-arginine-induced acute necrotizing pancreatitis in mice by enhancing SIRT1-mediated deacetylation of p53 and heat shock factor1. Int J Mol Med, 40(2): 427-437.
WATERFORD SD, KOLODECIK TR, THROWER EC, GORELICK FS (2005) Vacuolar ATPase regulates zymogen activation in pancreatic acini. J Biol Chem, 280(7): 5430-5434.
WHITAKER JF (1973) A rapid and specific method for the determination of pancreatic lipase in serum and urine. Clin Chim Acta, 44: 133-138.
ZIELINSKA D, KOLOZYN-KRAJEWSKA D (2018) Food-origin lactic acid bacteria may exhibit probiotic properties. Biomed Res Int, 2018: 5063185.