Acetaminophen is a commonly used analgesic and antipyretic drug that could exert acute and chronic liver cell failure. Mesenchymal stem cell therapy offers advantages for tissue repair and regeneration in clinical research. To elucidate the therapeutic effect of stem cells on different doses of acetaminophen-induced hepatotoxicity in adult male albino rats, sixty adult male albino rats weighing 170-230 g were divided into six groups: Group I (Normal control) were injected intraperitoneally with isotonic saline three times over one week. Group II receive a single intravenous injection of MSC. Group III and IV: were injected intraperitoneally three times over one week by acetaminophen at a dose of 300 mg/kg (Group III) and 600 mg/kg (Group IV) respectively. Group V and VI were injected with acetaminophen 300 mg/kg (Group IV) and 600 mg/kg (Group V), followed in both groups by a single intravenous injection of MSC 24 hours after the last dose of acetaminophen. Blood samples were collected from the tail vein of the rats for assessment of biochemical markers of liver, then they were anaesthetized and sacrificed. Liver homogenate was assessed for Malondialdehyde (MDA) and Super Oxide Dismutase (SOD). Liver specimens were subjected to histopathological examination and ultrastructural study. Statistical analysis was done for the results of hepatic biomarkers. Examination of specimens of group III and IV revealed disturbed hepatic architecture, nuclear and cytoplasmic degeneration and increased serum liver enzymes compared to the control group I. However, MSC markedly ameliorated these histological and laboratory alterations in both group V and VI. It could be concluded that stem cells had a beneficial role in treatment of hepatotoxicity exerted by acetaminophen administration in adult male albino rats.
Evaluation of the therapeutic role of stem cells in experimental acetaminophen induced hepatotoxicity in adult male albino rat
Lamya A. Abd Elsalam 1,2, Shereen E. Tawfeek 1,3, Eman H. Nadwa 4,5, Bahaa Eldin A. Khaled 1,6
1 Department of Anatomy, College of Medicines, Jouf University, Sakaka, Saudi Arabia
2 Department of Anatomy and Embryology, Faculty of Medicine, Beni Suef University, Egypt
3 Human anatomy and Embryology Department, Faculty of Medicine, Zagazig University, Egypt
4 Department of Pharmacology and Therapeutics, College of Medicines, Jouf University, Saudi Arabia
5 Department of Medical Pharmacology, Faculty of Medicines, Cairo University, Egypt
6 Department of Anatomy and Embryology, Faculty of Medicine, Cairo University, Egypt
SUMMARY
Sign up or Login
INTRODUCTION
Acetaminophen is an over-the-counter (OTC), commonly consumed pain killer and antipyretic; however, its safety is not absolute. High doses can cause acute and chronic liver cell failure and is correlated with significant increase in liver enzymes (Jarsiah et al., 2017).
High doses of acetaminophen leads to saturation of pathways in the liver responsible for its conjugation, shunting its metabolism to cytochrome P450 (CYP450) enzyme that results in the formation of a toxic metabolite, N-acetyl-p-benzoquinone imine (NAPQI). NAPQI itself is detoxified by conjugation with glutathione, but in case of acetaminophen overdose hepatocellular supplies of glutathione become depleted; NAPQI therefore accumulates and induces an inflammatory cascade leading to cell necrosis and apoptosis through direct mitochondrial damage (Larson, 2007; Jaeschke, 2012).
N-acetyl cysteine (precursor of glutathione) is the only therapeutic agent available for management of acetaminophen toxicity. The effectiveness of this antidote is time-related, in which it is only effective if given early in the course of toxicity. In cases of irreversible hepatic damage, liver transplantation is the last resort; however, this option is limited by availability of donors, costs, and complications of immunosuppression (Craig et al., 2012).
The value of mesenchymal stem cell (MSC) has been investigated in the field of tissue repair and regeneration. Potentially, MSC have the ability to replace injured tissues in the body, with minimal risk of adverse effects or rejection. Beside their ability to reside in injured organs, giving rise to subsequent generations with variable degrees of differentiation, MSC are postulated to convey immunomodulatory, anti-inflammatory and anti-fibrotic effects (Gurtner et al., 2007; Huang et al., 2016).
In treatment of acetaminophen toxicity, induction of liver regeneration by stem cell therapy is of potential value in improving outcomes (Bhushan et al., 2014). MSC convey hepatocyte-like markers, which make them a substantial alternative for liver transplantation (Scheers et al., 2011).
The aim of the present work is to evaluate the therapeutic effect of stem cells on different doses of acetaminophen-induced hepatotoxicity in adult male albino rats, and to assess the potential therapeutic role of MSC against that toxicity.
MATERIALS AND METHODS
Acetaminophen
It was supplied from Sigma Aldrich, dissolved in warm isotonic saline 0.9% and injected intraperitoneally (IP) to rats three times over one week at two different doses: 300 mg/kg (groups III and V) and 600 mg/kg (groups IV and VI) (Bhushan et al., 2014).
Bone marrow (BM)-derived mesenchymal stem cells (MSC)
MSC were obtained from the stem cell research unit at the Biochemistry Department of Kasr Alaini, Faculty of Medicine, Cairo University. Six-week-old male Sprague-Dawley rats were utilized to obtain the BM. Femora and tibiae of the animals were flushed with Dulbecco’s modified Eagle’s medium complemented by 10% fetal bovine serum. Afterwards, isolation of the nucleated cells and resuspension were carried out in complete culture medium complemented by 1% penicillin-streptomycin. Incubation of the cells was carried out at 37°C in 5% humidified CO2 for 12–14 days as primary culture or upon formation of large colonies. When they developed (80-90% confluence), twice wash of the cultures was done using PBS then cells were trypsinized with 0.25% trypsin in 1 mmol/l EDTA for 5 min at 37°C. Resuspension of the cells was done in serum-supplemented medium and incubated in a culture flask, after centrifugation. The formed culture was named as first-passage culture (Alhadlaq and Mao, 2004; Raafat et al., 2015).
Characterization of BM-derived MSCs
At harvest time, identification of MSC was achieved by detection of their adhesiveness or their spindle shape. By flow cytometric evaluation, MSC express CD29, one of surface markers of rat’s MSC (Payushina et al., 2006).
Labeling of stem cells with PKH26 dye
MSC were harvested during the 4th passage, and were labeled with PKH26 fluorescence linker dye (Sigma Aldrich, USA, Sigma brand, catalogue number: MINI 26), which was utilized for general cell-membrane labeling. It was used in the form of PKH26 dye stock solution (1 vial containing 0.1 ml, 1x10-3 M in ethanol) and diluent C (1 vial containing 10 ml), according to the manufacturer instructions for use. PKH26 is a red fluorochrome dye, which is stable and will divide equally when the cells divide. After staining with PKH dye, one can observe as many as eight divisions depending on how brightly the cells were stained initially and on the amount of surface area on the cells. Most commonly, 4-6 divisions can be visualized.
This dye was used in groups V and VI. The dose of MSC was 3x106 MSC in 0.5 ml phosphate buffer saline (PBS) (Kim et al., 2010), labeled with PKH26 dye, injected into the tail vein. Homing of the labelled cells in the liver was checked by fluorescent microscope (Sigma-Aldrich, Saint Louis, USA).
Animals and experimental design
Sixty adult male albino rats weighing 170-230 g were used in this study. They were obtained from the animal house, Faculty of Medicine, Cairo University. The experiment was carried out according to the standard guidelines of Institutional Animal Care and Use Committee and after Institutional Review Board approval. They were maintained under standard environmental and laboratory conditions with standard diet. The rats were divided equally into six groups, 10 rats each, as follows:
Group I (Control group): rats of this group were injected intraperitoneally three times over one week with one ml warm saline 0.9% (vehicle of acetaminophen). At day 7, they were injected with 0.5 ml of PBS (vehicle of MSC) intravenous, then they were sacrificed after one more week.
Group II (MSC treated group): rats of this group were injected intraperitoneally three times over one week with one ml warm saline 0.9% (vehicle of acetaminophen). At day 7, they received a single intravenous dose of MSCs (3 × 106 cells in 0.5 ml PBS), then they were sacrificed after one more week.
Group III (Acetaminophen 300 mg/kg group): rats were injected with acetaminophen at a dose of 300mg/kg, then they were sacrificed after one more week.
Group IV (Acetaminophen 600 mg/kg group): rats were injected with acetaminophen at a dose of 600 mg/kg, then they were sacrificed after one more week.
Group V (Acetaminophen 300 mg/kg+MSC group): rats were injected with acetaminophen at a dose of 300 mg/kg followed by a single intravenous injection of MSC, as group II, at day 7, then they were sacrificed after one more week.
Group VI (Acetaminophen 600 mg/kg+MSC group): rats were injected with acetaminophen at a dose of 600 mg/kg followed by a single intravenous injection of MSC, as group II, at day 7, then they were sacrificed after one more week.
At the end of the experiment, blood samples were obtained for assessment of biochemical markers of liver from all groups, then the rats were anaesthetized and sacrificed. Liver specimens were collected for preparation of tissue homogenate to detect oxidant/antioxidant markers, for light and electron microscopic study to evaluate histological structure and for fluorescence microscopic study to detect homing of mesenchymal stem cells.
Serum preparation
By the end of the experiment, under isoflurane anesthesia, blood samples were collected, under complete aseptic conditions, from the rat retro-orbital venous plexus by means of micro-capillary glass tubes. Blood was allowed to coagulate at room temperature, and then samples were centrifuged at 4000 rpm for 20 min using a cooling centrifuge (Sigma 3-30 k, USA). The clear serum layer was separated and stored at -80°C for assessment of Serum aspartate aminotransferase [AST], alanine aminotransferase [ALT] (Reitman and Frankel, 1957) and Gamma glutamyl transferase [GGT] (Lowryet al., 1951).
Tissue homogenate preparation for oxidant/antioxidant markers
Homogenous preparation of tissue for oxidant / antioxidant markers. The portion of excised liver tissue was homogenized in a buffer of 50 mmol / lTris-HCl (pH 7.4) and then centrifuged for 15 min at 10,000 g. Antioxidant status value at 4°C.
The resulting pellet was discarded and the supernatants were kept frozen immediately and then used for biochemical calculations. Determination of lipid peroxidation was done according to Ohkawa et al. (1979), as the formation of malondialdehyde (MDA) during an acid heating reaction. The activity of superoxide dismutase (SOD) was determined by measuring the inhibition of epinephrine autoxidation at pH 10.2 and 30°C, according to Misra and Fridovich (1972).
Histopathological assay
Rats of all groups were anesthetized with sodium thiopental 75 mg/kg (Gum and Cho, 2013). Liver specimens were carefully dissected, excised, prepared for histopathological examination and ultrastructural study.
Preparation of paraffin sections
Liver specimens were immediately fixed in 10% formalin solution in normal saline and embedded in paraffin. Blocks were prepared for sectioning at 4-5 microns. Sections of samples were collected on glass slides, deparaffinized, and stained by Hematoxylin and Eosin to show the general structure of the tissue, by Periodic Acid Schiff (PAS) to show the glycogen content and by Masson’s trichrome to visualize the deposition of collagen fibers distribution (Bancroft and Gamble, 2008). Slides were examined by the light electric microscope (LEICA ICC50 W), Faculty of Agriculture, Cairo University.
Ultrastructure preparations (Hayat, 1986)
Fixation of liver specimens was carried out using 2.5% glutaraldehyde for 24-48 h, followed by washing in phosphate buffer (pH 7.2-7.4) three to four times for 20 min. The specimens were then post-fixed in in a buffered solution of 1% osmium tetraoxide for 2 h, and after that washed in a similar buffer four times for 20 min each. Fixed specimens were dried out in increasing concentrations of ethyl alcohol (30%, half, 70%, 90%, and 100%), cleared in two changes of propylene oxide and inserted in Epon resin. The pitch squares underwent a process of trimming to get rid of the surplus tissue. Ultrathin sections (60-90 nm thick) and illustrative fields of semithin sections were chosen and were cut with a diamond knife using a Reichert OMVs ultra microtome, mounted on copper frameworks. These semithin sections were subjected to double staining with uranyl acetate and lead citrate (Weakley, 1981). The grids were examined and photographed using a transmission electron microscope (JEOL JEM-1200 EX II, Japan) operated at 60-70 kV, at (Electron Microscope Unit), pathology department, National Cancer Institute (NCI).
Statistical analysis
The collected data from liver biochemical markers were coded and analyzed by computer, using a data base software program. The collected data were organized, tabulated and statistically analyzed using SPSS software statistical computer package version 22 (SPSS Inc, USA). The mean and standard deviation were calculated. ANOVA (Analysis of variance) was used to test the difference of mean values of measured parameters among groups; multiple comparison between pairs of groups were performed using Tukey HSD (Post hoc range test). For interpretation of results, significance was adopted at P ≤ 0.05, while P-values of <0.01 indicate highly significant results. All the results were expressed as mean ± S.D.
RESULTS
Light microscopic results
Examination of rat liver specimens stained with haematoxylin and eosin of group I (Control group) and group II (MSC treated group) showed normal hepatic architecture; sections from these groups revealed mononucleated and binucleated hepatocytes radiating from the central vein, and hepatic sinusoids lined with flattened endothelial cells. Kupffer cells could also be seen (Fig. 1A). The portal triad was seen formed of bile ductule lined with cuboidal or low columnar epithelium, a branch of the portal vein exhibiting a thin wall and wide lumen, as well as branches of the hepatic artery characterized by a thicker wall and narrower lumen (Fig. 1B).
The hepatic structure was moderately disturbed in group III (Acetaminophen 300 mg/kg group), where the central vein was engorged and dilated; the hepatic sinusoids also appeared engorged. Moreover, hepatocytes exhibited cytoplasmic vacuolation, pyknotic nuclei and karyolysis (Fig. 1C). Also, there was increase in Kupffer cells, engorged portal vein, proliferated bile ductules and thick hypertrophied hepatic artery (Fig. 1D). Examination of group IV (Acetaminophen 600 mg/kg group) exhibited marked disturbance of hepatic parenchymal architecture in the form of markedly congested central vein, extravasated blood (Fig. 1E), markedly congested or hypertrophied portal vein, thick hypertrophied hepatic artery and mononuclear cell infiltration around proliferated bile ductules (Figs. 1F, G). Moreover, most hepatocytes exhibited extensive fat degeneration, nuclear pyknosis and karyolysis, and cytoplasmic vacuolation (Figs. 1E, F, G).
Examination of sections from group V (Acetaminophen 300 mg/kg+MSC group) showed more or less normal parenchymal architecture; most sinusoids and hepatocytes were radiating from the central vein, although few hepatocytes exhibited pyknotic and karyolitic nuclei. Mild increase of Kupffer cells could also be seen (Figs. 2A, B). Examination of portal area revealed apparently normal hepatocytes around components of portal tract (Fig. 2C). While in group VI (Acetaminophen 600 mg/kg+MSC group) the cells showed mildly disturbed parenchymal architecture, most sinusoids and hepatocytes were apparently normal and radiating from the central vein, which appeared either normal (Fig. 2D) or markedly congested and surrounded by mononuclear cellular infiltration (Fig. 2E), markedly hypertrophied portal vein, hepatic artery and marked proliferation of bile ductules (Fig. 2F). Some hepatocytes exhibited extensive fat degeneration, cytoplasmic vacuolation, pyknotic and karyolitic nuclei. Marked increase in Kupffer cells could also be seen (Figs. 2D, E, F).
In sections stained with PAS, the hepatocytes appeared to have a strong positive PAS reaction in the form of small red granules filling their cytoplasm, denoting considerable amount of glycogen in control group I and II (Fig. 3A) while these reactions gradually decrease in both group III and VI respectively, which showed moderate positive reaction in group III (Fig. 3B) and faint reaction in group IV (Fig. 3C). Group V restored the strong reaction again (Fig. 3D), while group VI exhibited a moderate one (Fig. 3E).
Sections stained with Masson’s trichrome demonstrated a thin layer of collagen fibers around the central vein and adjoining hepatic sinusoids, while there was a small amount of it surrounding the elements of the portal triad in control group I and group II (Figs. 4A, B). On the contrary, group III sections demonstrated moderate increase of these collagen depositions (Figs. 4C, D), and marked increase in group IV (Figs. 4E, F). Moreover, both groups V (Figs. 5A, B) and VI (Figs. 5C, D) exhibited this noticeable collagen deposition.
Electron microscopic results
Electron microscopic examination of the control group I and MSC treated group II revealed normal structure of hepatocytes, where there was normal rough endoplasmic reticulum and spherical to ovoid mitochondria with intact cristae. The nucleus was surrounded by bilaminar envelope with nuclear pores, and displayed dispersed chromatin with a prominent nucleolus. Golgi apparatus could also be observed opposite nuclear pores (Fig. 6A). Examination of cells of group III revealed apparently normal nucleus with intact nuclear envelop, rough endoplasmic reticulum with maintained ribosomal surface and cytoplasmic rarefaction. Most of mitochondria were apparently normal, with intact cristae, and few of them were ballooned with absent cristae (Fig. 6B), while in group IV there was extensive damage of most of intracellular organelles with irregular and extremely shrunken nuclei with clumped chromatin material, indented nuclear envelope, extensive cytoplasmic rarefaction, fragmented rough endoplasmic reticulum with lost ribosomal surface. Moreover, the mitochondria were mostly ballooned with absent cristae and disrupted membranes. Few mitochondria and rough endoplasmic reticulum were apparently normal (Figs. 6C, D), while in group V there was slight restoration of normal structure of the cells, which showed apparently normal nucleus, dispersed chromatin, prominent nucleoli, intact nuclear envelope and Golgi apparatus opposite nuclear pores. Abundant mitochondria with normal cristal pattern were also detected with mild cytoplasmic rarefaction (Fig. 6E). Group VI also demonstrated mild return of the normal cellular structure, where the nucleus appeared with fairly clumped chromatin material, prominent nucleolus and indented nuclear envelope, apparently normal rough endoplasmic reticulum with its ribosomal surface and few areas of cytoplasmic rarefaction. Most of mitochondria appeared normal and few of them were ballooned with damaged cristae and disrupted membranes (Fig. 6F).
Labeling of stem cells with PKH26
In group V and VI, the MSCs labeled with PKH26 fluorescent dye were detected in the examined hepatic tissue, confirming homing of these cells into the liver (Fig. 7A, B).
Serum biochemical markers results
Serum liver biochemical markers (AST, ALT and GGT) showed statistically significant differences in group III, IV and VI compared to group I (p <0.0001). There was a statistically significant difference between the group III and group IV. AST, ALT and GGT were significantly lower in group V compared to group III and in group VI compared to group IV. Moreover, there was a statistically significant difference between the group V and group VI (p = 0.004). However, there was no statistically significant difference between the control and group V (p = 0.657) (Figs. 8A, B, C).
Liver oxidant/antioxidant markers results
Liver homogenate levels of MDA showed statistically significant difference in group III, IV and VI compared to group I (p <0.0001). There was a statistically significant difference between the group III and group IV. MDA was significantly lower in group V compared to group III, and in group VI compared to group IV. Moreover, there was a statistically significant difference between the group V and group VI (p = 0.004) However, there was no statistically significant difference between the control and group V (p = 0.842) (Fig. 9A).
Liver homogenate levels of SOD showed statistically significant difference in group III, IV and VI compared to group I (p <0.0001). There was a statistically significant difference between the group III and group IV. SOD was significantly higher in group V compared to group III and in group VI compared to group IV. Moreover, there was a statistically significant difference between the group V and group VI (p = 0.004). However, there was no statistically significant difference between the control and group V (p = 0.612) (Fig. 9B).
DISCUSSION
Although acetaminophen is widely used for its analgesic and antipyretic actions, its overdose is also associated with hepatotoxicity (Wootton and Lee, 1990; Zimmerman and Maddrey, 1995; Lee, 2013). The present study demonstrated the effects of this toxicity, as well as the therapeutic values of MSCs to alleviate these effects.
In the present work, manifestations of the pathological impact of two different doses of acetaminophen on the rat liver were recorded in group III and group IV; 300 mg/kg and 600 mg/kg respectively, with marked histological and laboratory alterations in group IV. Light microscopic examination revealed disrupted hepatic parenchymal architecture, increased number of Kupffer cells, inflammatory cellular infiltration, dilatation and congestion of the central, portal veins and hepatic sinusoids, proliferated bile ductules, extravasated blood, hepatocytic degeneration in the form of nuclear pyknosis, karyolysis, depletion of glycogen content, cytoplasmic vacuolation and fatty degeneration. The biochemical study showed markedly elevated liver enzymes especially in high dose (600 mg/kg). The electron microscopic examination presented thick plasma membrane, cytoplasmic degeneration, rarefaction and fat degeneration, increased electron dense lysosomes, ballooning of mitochondria, fragmented rough endoplasmic reticulum and degenerated nucleus with clumped chromatin material. These results could be attributed to the increased oxidative stress evidenced in groups III and IV by the significant increased level of MDA, indicating high level of the noxious reactive oxygen species (ROS), as well as the decreased level of the antioxidant enzyme SOD. These findings were in accordance with previous studies, which reported that large doses of acetaminophen lead to excessive formation of NAPQI, which triggers cell damage via an imbalance in oxidative stress involving high levels of ROS, such as hydroxyl radicals (OH), superoxide (O2−) and peroxy nitrite, with low levels of antioxidant enzymes, such as glutathione peroxidase and superoxide dismutase, eventually causing acute liver cell injury and cell death (Kay et al., 2011; McGill, 2012; Gum and Cho, 2013; Kane, 2018).
In the present work, mitochondrial and rough endoplasmic reticulum damage was evident in rats given acetaminophen alone (groups III and IV), which could be accredited to the imbalanced oxidative stress. This was in agreement with Jaeschke et al., 2014 and Du et al., 2017), who attributed these findings to reactive oxygen species that directly injure the mitochondria, followed by disturbance of calcium homeostasis and protein synthesis with subsequent cell necrosis.
In the current study, acetaminophen administration was associated with marked increase in collagen fibers deposition. These findings were in agreement with Bai et al. (2017) and Alwahsh et al. (2019), who recorded hepatic fibrotic changes following repeated doses of acetaminophen. The mechanism of fibrosis was explained by Friedman (2008), who pointed out that the main cell responsible for fibrogenesis is the hepatic stellate cell which produces type I and type IV collagen. In case of injury, it undergoes activation, characterized by cellular proliferation with increased endoplasmic reticulum, increased contractility with prominent smooth muscle specific α-actin, increased secretion of cytokines and eventually fibrosis.
In the present work, groups III and IV also showed marked elevation in liver enzymes denoting marked liver cell damage, which could be also explained by ROS resulting from acetaminophen administration. Similar findings were found by Dart and Baily (2007), who mentioned that one gram of acetaminophen four times a day can cause elevation of liver enzymes three times the normal values. It was reported that administration of acetaminophen leads to marked increase in serum liver enzymes and decrease in serum albumin level with consequent liver cell failure.
Thuwaini et al. (2016) and Khorsandi et al. (2010) reported that acetaminophen leads to marked elevation of serum liver enzymes after one hour and three hours following administration, and added that evaluation of serum ALT in the early hours can be more helpful than AST level. Jarsiah et al. (2017) concluded that acetaminophen caused hepatic injury resulting from cell membrane and mitochondrial damage, and this was manifested by elevation of serum ALT and AST. Furthermore, GGT is released extensively from damaged hepatic cells to the blood stream, and hence it has a diagnostic value in hepatobiliary diseases. Such data were similar to the findings of the present research.
In the current study, MSC therapy markedly ameliorated the histological and biochemical alterations exerted by acetaminophen administration in groups V and VI, which could be attributed to the improvement of oxidative stress parameters in the form of significant decreased MDA and significant increased SOD levels in these groups. These findings were in accordance with Ayatollahi et al. (2014), who demonstrated the therapeutic role of MSC in case of hepatic injury caused by the oxidative stress of carbon tetrachloride. Moreover, Zeng et al. (2015) also reported the augmented therapeutic effect of MSC with intensified antioxidant power in the treatment of acute hepatic failure.
Previous studies (Huang et al., 2016; Wang et al., 2018) illustrated another mechanism underlying the therapeutic role of MSC in the form of the immunosuppression that downregulates proinflammatory cytokines (IL-1α, IL-1β) and upregulates anti-inflammatory cytokines (IL-6, IL-10). In addition, MSC improves liver function and increases expression of growth factors and cytokines that guide MSC home in the injured tissue, which promotes hepatic regeneration and restoration of normal liver functions. Moreover, MSC can differentiate into mature hepatocytes, with restoration of their structural and functional activity, regaining the normal hepatic parenchymal architecture. Vassilopoulos et al. (2003) and Milosavljevic et al. (2017) hypothesized that conversion of MSCs to hepatocytes may occur via cell fusion. Bystander effect is probably due to factors secreted by MSC that direct them to the site of injury, leading to increased mitotic activity of hepatocytes. The release of vascular endothelial growth factor from stem cells increasing the blood supply is another theory to explain the regeneration of injured liver cells and improvement in liver functions (Tang et al., 2006). Furthermore, MSC may act by overexpression of the Bcl-2 gene inhibiting apoptosis (Chen et al., 2001) or by suppression of interleukin-6 (IL-6) pathway inhibiting inflammation (Wang et al., 2006). Lastly, tissue-specific stem cells, such as oval cells in the liver may be stimulated by hepatic stellate cells enhancing the regeneration of the injured tissue (Austin and Lagasse, 2003).
In the current study, liver fibrosis was attenuated after administration of MSC. This could be explained by the theory that MSC express certain antifibrotic factors, such as matrix metalloproteinase 9 (MMP-9) and release some factors, such as soluble Kit- ligand related to their proliferation and differentiation (Kallis et al., 2007; Segovia-Silvestre et al., 2011). It was concluded that after 4 weeks of MSC transplantation, significant decrease of alpha smooth muscle specific α-actin occurred. Thus, transplanted MSC may inhibit activated stellate cells by them or guide them to apoptosis (Ezzat et al., 2012). Contradicting the observed data of the present study, Di Bonzo et al. (2008) and Yan et al. (2009) have demonstrated that MSC display a profibrogenic propensity. Indeed, intravenously infused umbilical cord MSC contributed to the myofibroblast population (smooth muscle specific α-actin and fibroblast secretory protein-1 positive cells) in liver injury.
MSC administration markedly decreased serum liver enzymes ALT, AST and GGT, which could be attributed to improved oxidative stress markers evidenced by significant increased SOD and significant decreased MDA levels. This was in accordance with Zhao et al. (2012), who mentioned that MSC injection causes marked decrease in serum levels of liver enzymes and serum bilirubin, and the results were better with early treatment due to restoration of normal cellular and functional pattern of hepatocytes as a result of MSC hepatic differentiation into hepatocytes. Kuo et al. (2008), Song et al. (2015) and Idriss et al. (2018) demonstrated that the effectiveness of stem cell therapy depends greatly on the route of transplantation. They revealed that intravenous MSC-derived hepatocyte transplantation in rescuing liver failure was superior to intrasplenic, intraperitoneal, or even intrahepatic transplantation.
Conclusion
The overdose of acetaminophen results in deleterious effects on the liver, which could be detected in the present work on the structure and biochemical aspects. MSC executed significant improvement of these harmful effects evidenced by homing of MSC in liver and improvement at both structural and functional (confirmed by biochemical results of oxidative stress markers) levels.
Related articles
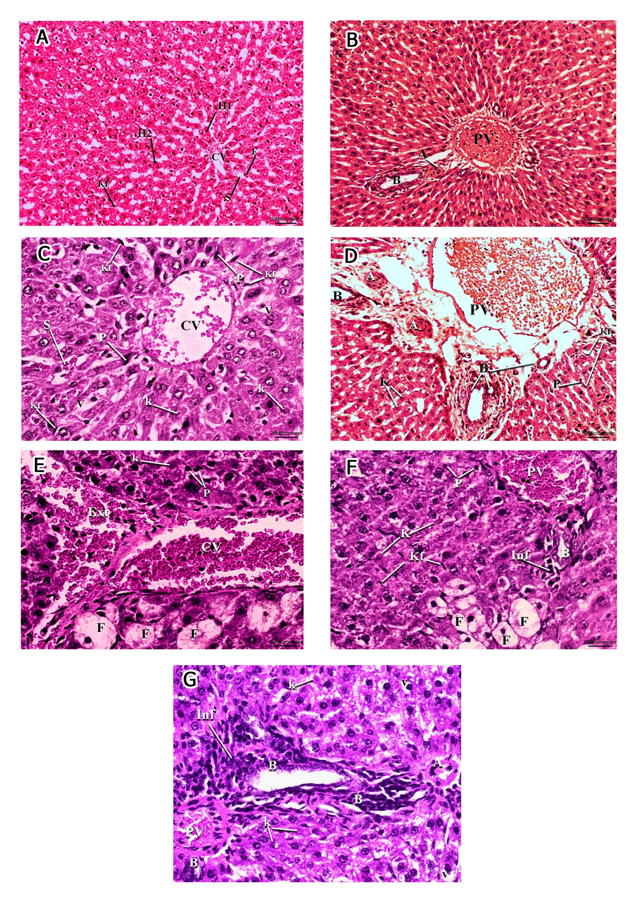



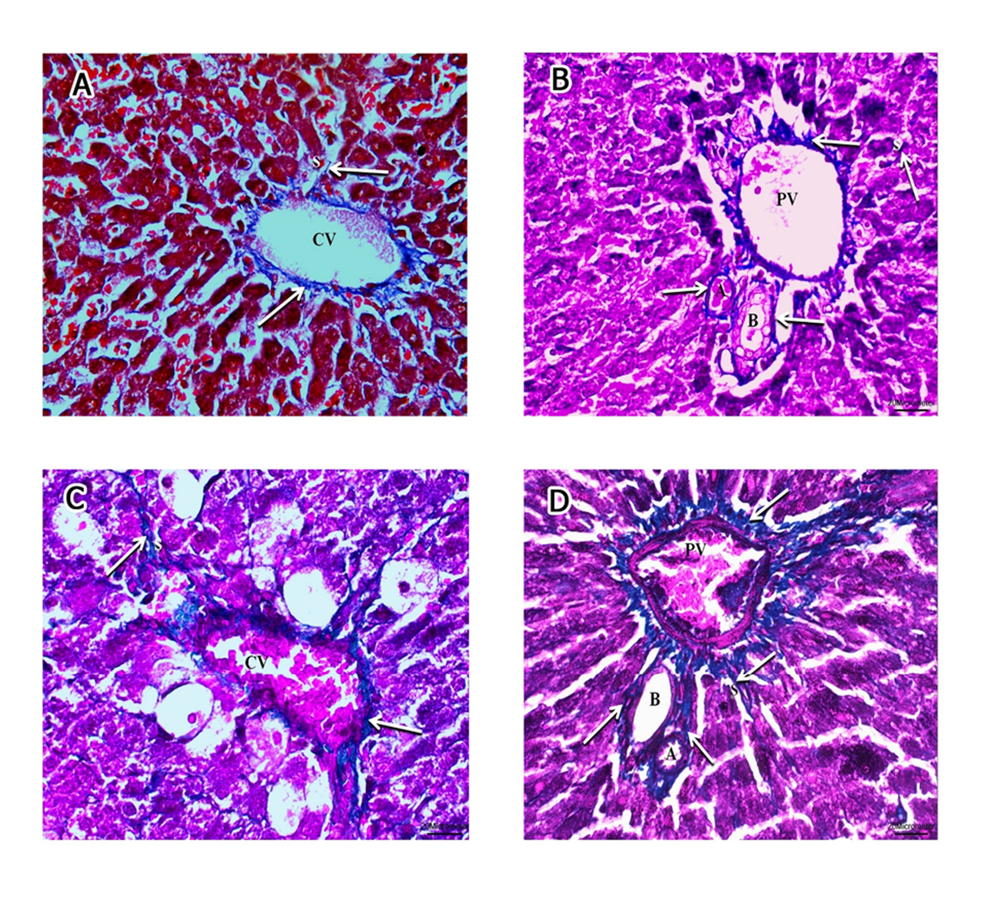
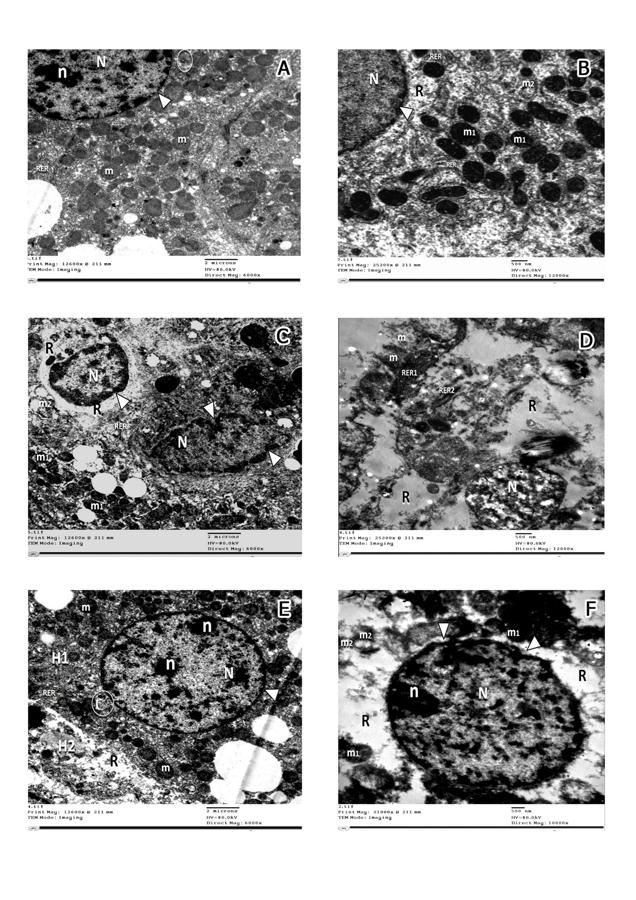


ALHADLAQ A, MAO JJ (2014) Mesenchymal stem cells: isolation and therapeutics. Stem Cells Dev, 13: 436-448.
ALWAHSH M, OTHMAN A, HAMADNEH L, TELFAH A, LAMBERT J, HIKMAT S, ALASSI A, MOHAMED F, HERGENRÖDER R, AL-QIRIML T, DOOLEY S, HAMMAD S (2019) Second exposure to acetaminophen overdose is associated with liver fibrosis in mice. EXCLI J, 18: 51-62.
AUSTIN TW, LAGASSE E (2003) Hepatic regeneration from hematopoietic stem cells. Mech Dev, 120(1): 131-135.
AYATOLLAHI M, HESAMI Z, JAMSHIDZADEH A, GRAMIZADEH B (2014) Antioxidant effects of bone marrow mesenchymal stem cell against carbon tetrachloride-induced oxidative damage in rat livers. Int J Org Transplant Med, 5(4): 166-173.
BAI Q, YAN H, SHENG Y, JIN Y, SHI L, JI L, WANG Z (2017) Long-term acetaminophen treatment induced liver fibrosis in mice and the involvement of Egr-1. Toxicology, 382: 47-58.
BANCROFT JD, GAMBLE M (2008) Connective tissue stains. In: Bancroft J (ed). Theory and Practice of Histological Techniques. Churchill-Livingston Elsevier, London, pp 135-160.
BHUSHAN B, WALESKY C, MANLEY M, GALLAGHER T, BORUDE P, EDWARDS G, MONGA SP, APTE U (2014) Pro-regenerative signaling after acetaminophen-induced acute liver injury in mice identified using a novel incremental dose model. Am J Pathol, 184(11): 3013-3025.
CHEN Z, CHUA CC, HO YS, HAMDY RC, CHUA BH (2001) Overexpression of Bcl-2 attenuates apoptosis and protects against myocardial I/R injury in transgenic mice. Am J Physiol Heart Circ Physiol, 280(5): H2313-H2320.
CRAIG DG, BATES CM, DAVIDSON JS, MARTIN KG, HAYES PC, SIMPSON KJ (2012) Staggered overdose pattern and delay to hospital presentation are associated with adverse outcomes following paracetamol-induced hepatotoxicity. Br J Clin Pharmacol, 73(2): 285-294.
DART RC, BAILEY E (2007) Does therapeutic use of acetaminophen cause acute liver failure? Pharmacotherapy, 27(9): 1219-1230.
DI BONZO LV, FERRERO I, CRAVANZOLA C, MARESCHI K, RUSTICHELL D, NOVO E, SANAVIO F, CANNITO S, ZAMARA E, BERTERO M, DAVIT A (2008) Human mesenchymal stem cells as a two-edged sword in hepatic regenerative medicine: engraftment and hepatocyte differentiation versus profibrogenic potential. Gut, 57(2): 223-231.
DU K, FARHOOD A, JAESCHKE H (2017) Mitochondria-targeted antioxidant Mito-Tempo protects against acetaminophen hepatotoxicity. ArchToxicol, 91: 761-773.
EZZAT T, DHAR DK, MALAGO M, DAMINK SWO (2012) Dynamic tracking of stem cells in an acute liver failure model. World J Gastroenterol, 18(6): 507-516.
FRIEDMAN SL (2008) Mechanisms of hepatic fibrogenesis. Gastroenterology, 134(6): 1655-1669.
GUM SI, CHO MK (2013) Recent updates on acetaminophen hepatotoxicity the role of nrf2 in hepatoprotection. Toxicol Res, 29(3): 165-172.
GURTNER GC, CALLAGHAN MJ, LONGAKER MT (2007) Progress and potential for regenerative medicine. Annu Rev Med, 58: 299-312.
HAYAT A (1986) Principles of tissue preparation for electron microscopy. In: Hayat MA (ed). Basic techniques for transmission electron microscopy, 1st edn. Macmillan Press, New York, pp 22-46.
HUANG YJ, CHEN P, LEE CY, YANG SY, LIN MT, LEE HS, WU YM (2016) Protection against acetaminophen-induced acute liver failure by omentum adipose tissue derived stem cells through the mediation of Nrf2 and cytochrome P450 expression. J Biomed Sci, 23(1): 5.
IDRISS NK, SAYED HG, OSAMA A, SABRY D (2018) Treatment efficiency of different routes of bone marrow-derived mesenchymal stem cell injection in rat liver fibrosis model. Cell Physiol Biochem, 48(5): 2161-2171.
JAESCHKE H, MCGILL MR, RAMACHANDRAN A (2012) Oxidant stress, mitochondria, and cell death mechanisms in drug-induced liver injury: lessons learned from acetaminophen hepatotoxicity. Drug Metab Rev, 44(1): 88-106.
JAESCHKE H, XIE Y, MCGILL MR (2014) Acetaminophen-induced liver injury: from animal models to humans. J Clin Transl Hepatol, 2(3): 153-161.
JARSIAH P, NOSRATI A, ALIZADEH A, HASHEMI-SOTEH SMB (2017) Hepatotoxicity and ALT/AST enzymes activities change in therapeutic and toxic doses consumption of acetaminophen in rats. International Biol Biomed J, 3(3): 119-124.
KALLIS YN, ALISON MR, FORBES SJ (2007) Bone marrow stem cells and liver disease. Gut, 56(5): 716-724.
KANE AE, MITCHELL SJ, PAJKOS JM, MCKENZIE C, JONES B, COGGER V, COUTEUR DGL, CABOD R, HILMER SN (2018) Acetaminophen hepatotoxicity in mice: Effect of age, frailty and exposure type. Exp Gerontol, 73: 95-106.
KAY HY, KIM YW, RYU DH, SUNG SH, HWANG SJ, KIM SG (2011) Nrf2-mediated liver protection by sauchinone, an antioxidant lignan, from acetaminophen toxicity through the PKCδ-GSK3β pathway. Br J Pharmacol, 163(8): 1653-1665.
KHORSANDI LS, JAVADNIA F, ORAZIZADEH M, ABDOLAHI M (2010) Effect of green tea (Camellia sinensis L.) extract on acetaminophen induced acute hepatotoxicity in mice. Iranian J Med Arom Plants, 26(1): 22-29.
KIM TH, KIM JK, SHIM W, KIM SY, PARK TJ, JUNG JY (2010) Tracking of transplanted mesenchymal stem cells labeled with fluorescent magnetic nanoparticle in liver cirrhosis rat model with 3-T MRI. Magn Reson Imaging, 28(7): 1004-1013.
KUO TK, HUNG SP, CHUANG CH, CHEN CT, SHIH YRV, FANG SCY, YANG VW, LEE OK (2008) Stem cell therapy for liver disease: parameters governing the success of using bone marrow mesenchymal stem cells. Gastroenterology, 134(7): 2111-2121.
LARSON AM (2007) Acetaminophen hepatotoxicity. Clin Liver Dis, 11(3): 525-548.
LEE WM (2013) Drug-induced acute liver failure. Clin Liver Dis, 17(4): 575-586.
LOWRY OH, ROSEBROUGH NJ, FARR AL, RANDALL RJ (1951) Protein measurement with the folin phenol reagent. J Biol Chem, 193: 265-275.
MCGILL MR, WILLIAMS CD, XIE Y, RAMACHANDRAN A, JAESCHKE H (2012) Acetaminophen-induced liver injury in rats and mice: comparison of protein adducts, mitochondrial dysfunction, and oxidative stress in the mechanism of toxicity. Toxicol Appl Pharmacol, 264(3): 387-394.
MILOSAVLJEVIC N, GAZDIC M, SIMOVIC MARKOVIC B, ARSENIJEVIC A, NURKOVIC J, DOLICANIN Z, DJONOV V, LUKIC ML, VOLAREVIC V (2017) Mesenchymal stem cells attenuate acute liver injury by altering ratio between interleukin 17 producing and regulatory natural killer T cells. Liver Transplant, 23(8): 1040-1050.
MISRA HP, FRIDOVICH I (1972) The role of superoxide anion in the autoxidation of epinephrine and a simple assay for superoxide dismutase. J Biol Chem, 247(10): 3170-3175.
OHKAWA H, OHISHI N, YAGI K (1979) Assay for lipid peroxides in animal tissues by thiobarbituric acid reaction. Anal Biochem, 95(2): 351-358.
PAYUSHINA OV, DOMARATSKAYA EI, STAROSTIN VI (2006) Mesenchymal stem cells: sources, phenotype, and differentiation potential. Biol Bull, 33: 2-18.
RAAFAT N, ABDEL AAL SM, ABDO FK, EL GHONAIMY NM (2015) Mesenchymal stem cells: in vivo therapeutic application ameliorates carbon tetrachloride induced liver fibrosis in rats. Int J Biochem Cell Biol, 68: 109-118.
REITMAN S, FRANKEL S (1957) A colorimetric method for the determination of serum glutamic oxalacetic and glutamic pyruvic transaminases. Am J Clin Pathol, 28(1): 56-63.
SCHEERS I, LOMBARD, NAJIMI M, SOKA EM (2011) Cell therapy for the treatment of metabolic liver disease: an update on the umbilical cord derived stem cells candidates. Open Tissue Eng Regen Med J, 4: 48-53.
SEGOVIA-SILVESTRE T, REICHENBACH V, FERNÁNDEZ-VARO G, VASSILIADIS E, BARASCUK N, MORALES-RUIZ M, KARSDAL MA, JIMÉNEZ W (2011) Circulating CO3-610, a degradation product of collagen III, closely reflects liver collagen and portal pressure in rats with fibrosis. Fibrogenesis Tissue Repair, 4(1): 19.
SONG YM, LIAN CH, WU CS, JI A, XIANG JJ, WANG XY (2015) Effects of bone marrow derived mesenchymal stem cells transplanted via the portal vein or tail vein on liver injury in rats with liver cirrhosis. Exp Therap Med, 9(4): 1292-1298.
TANG J, XIE Q, PAN G, WANG J, WANG M (2006) Mesenchymal stem cells participate in angiogenesis and improve heart function in rat model of myocardial ischemia with reperfusion. Eur J Cardio-Thoracic Surg, 30(2): 353-361.
THUWAINI MM, KADHEM HS, HATEM HA (2016) Reducing the effects of paracetamol-induced hepatic damage in rat with fluvastatin drug. Univ Thi-Qar J, 11(4): 143-161.
VASSILOPOULOS G, WANG PR, RUSSELL DW (2003) Transplanted bone marrow regenerates liver by cell fusion. Nature, 422(6934): 901.
WANG M, TSAI BM, CRISOSTOMO PR, MELDRUM DR (2006) Pretreatment with adult progenitor cells improves recovery and decreases native myocardial proinflammatory signaling after ischemia. Shock, 25(5): 454-459.
WANG YH, WU DB, CHEN B, CHEN EQ, TANG H (2018) Progress in mesenchymal stem cell-based therapy for acute liver failure. Stem Cell Res Therapy, 9(1): 1-9.
WEAKLEY BS (1981) A beginner’s handbook in biological transmission electron microscopy. Ed. Churchill Livingstone; 2nd ed.
WOOTTON FT, LEE WM (1990) Acetaminophen hepatotoxicity in the alcoholic. South Med J, 83(9): 1047-1049.
YAN Y, XU W, QIAN H, SI Y, ZHU W, CAO H, ZHOU H, MAO F (2009) Mesenchymal stem cells from human umbilical cords ameliorate mouse hepatic injury in vivo. Liver Int, 29(3): 356-365.
ZENG W, XIAO J, ZHENG G, XING F, TIPOE G, WANG X, HE C, CHEN Z, LIU Y (2015) Antioxidant treatment enhances human mesenchymal stem cell anti-stress ability and therapeutic efficacy in an acute liver failure model. Sci Rep, 5: 11100.
ZHAO W, LI JJ, CAO DY, LI, ZHANG LY, HE Y, YUE SQ, WANG DS, DOU KF (2012) Intravenous injection of mesenchymal stem cells is effective in treating liver fibrosis. World J Gastroenterol, 18(10): 1048-1058.
ZIMMERMAN HJ, MADDREY WC (1995) Acetaminophen (paracetamol) hepatotoxicity with regular intake of alcohol: analysis of instances of therapeutic misadventure. Hepatology, 22(3): 767-773.